Global detection of keratoconus in its earliest stages remains a challenge to our field. However, increased adoption of corneal topography and, more recently, tomography has improved detection rates at incipient stages, even when overt clinical findings may be absent or mild. In turn, there has been great variability in estimates of disease prevalence around the world, with many countries now reporting a higher prevalence than previous thought. In Saudi Arabia, for instance, one study has reported the prevalence of keratoconus as 4,790 per 100,000 adolescents,1 whereas in Russia the prevalence was closer to 0.2-0.4 per 100,000.2 With the demonstrated ability of procedures such as corneal crosslinking to halt ectatic progression, a global initiative towards keratoconus screening and early detection is critical to improving long-term prognosis.
This early identification, especially when coupled with therapies that aim to prevent progression, may forestall the need for corneal transplantation.3 While topography and tomography remain the gold standard for diagnosing keratoconus today, alternative modalities now exist. These tools can increase diagnostic certainty in borderline cases and improve global surveillance efforts. Let’s review the tools we have at our disposal for that essential project.
TOPOGRAPHY AND TOMOGRAPHY
Nowadays, 3D digital corneal imaging is the mainstay diagnostic for detecting keratoconus.4 While corneal topography (eg, Placido disc reflection) measures anterior corneal power and may aid in detection of manifest keratoconus, tomography (eg, Scheimpflug imaging, slit scanning or optical coherence tomography [OCT]) has the additional ability to measure changes in posterior corneal curvature. Numerous studies have demonstrated that posterior corneal elevation may be one of the earliest detectable signs of keratoconus (Figure 1),5,6 and a global consensus of experts has determined that abnormal posterior elevation is a sine qua non for diagnosing subclinical keratoconus.7
Built-in software can aid interpretation of tomographic data by calculating multivariate screening indices (eg, Belin/Ambrósio enhanced ectasia display total derivation [BAD-D]), which provide a gestalt of the entire corneal shape, allowing the provider to quickly screen for ectasia (Figure 1).4 With the increasing use of both anterior and posterior corneal measurements in screening, further work is needed to standardize a new classification system that can diagnose early keratoconus based on both topographic and tomographic criteria.3 Additionally, the task of screening children, developmentally delayed individuals or patients in remote areas using this modality remains a challenge.3 Portable topography, such as the EyeSys Vista device (EyeSys Vision LLC), is now available and holds promise for expanding access to care to these populations.3
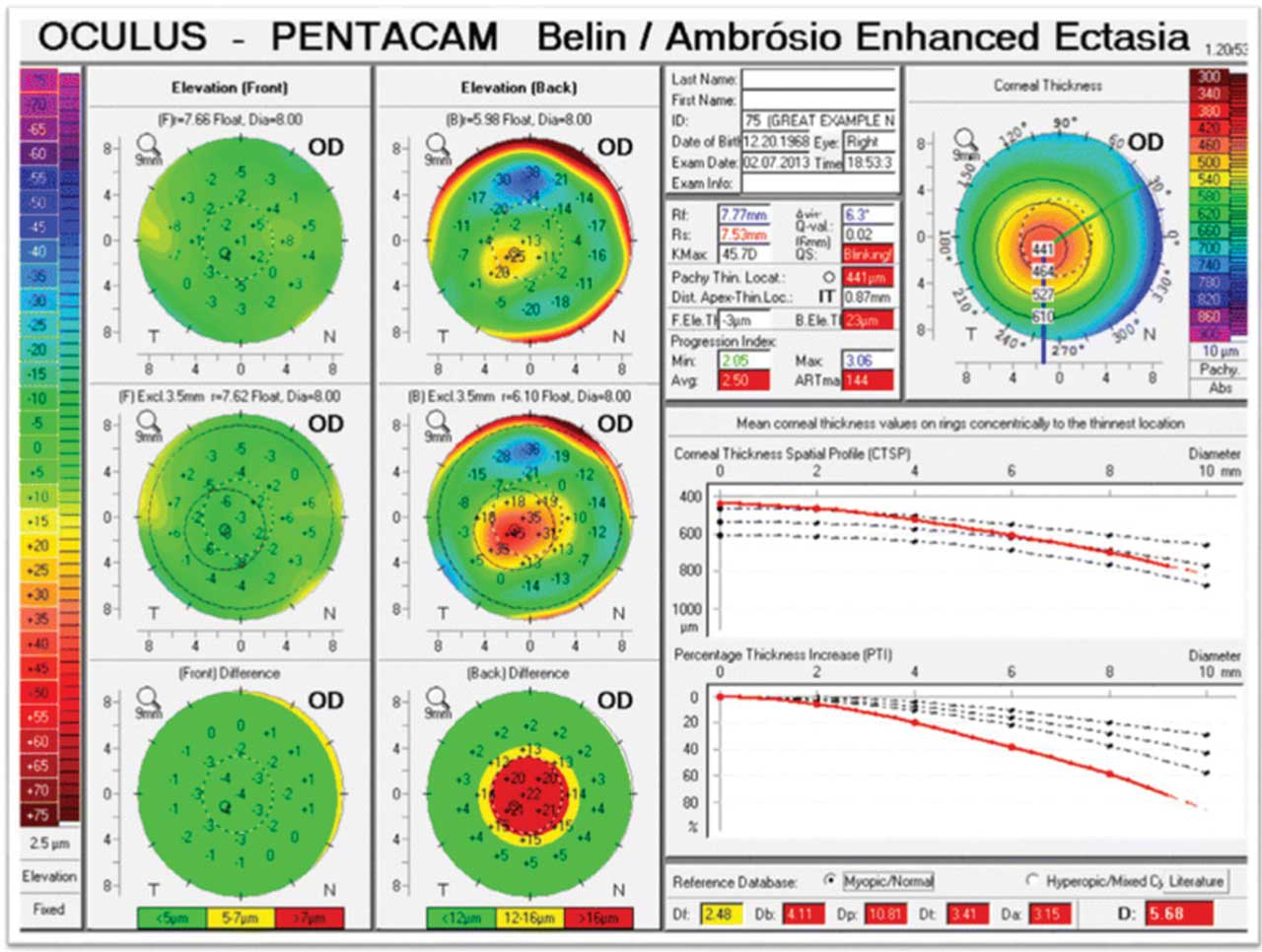
EPITHELIAL THICKNESS MAPPING
In contrast to central corneal thickness, which has been found to be a poorly reliable metric in diagnosing keratoconus,7 recent studies have demonstrated the utility of an anterior segment OCT-based epithelial thickness map in diagnosing subclinical keratoconus.8 The epithelium of keratoconic eyes typically thins at the corneal apex, in the area of the cone, and thickens circumferentially around it (Figure 2).4 In addition, there may be thinning and breakdown of Bowman’s layer and inferotemporal thinning of the corneal stroma.4 Using an OCT-based epithelial thickness profile has demonstrated a 96% sensitivity and 100% specificity for differentiating eyes with subclinical ectasia from normal.8
Limitations include poor OCT segmentation and decreased measurement accuracy when imaging keratoconic corneas.4 Nevertheless, thickness mapping has the potential to be an important adjunctive diagnostic.
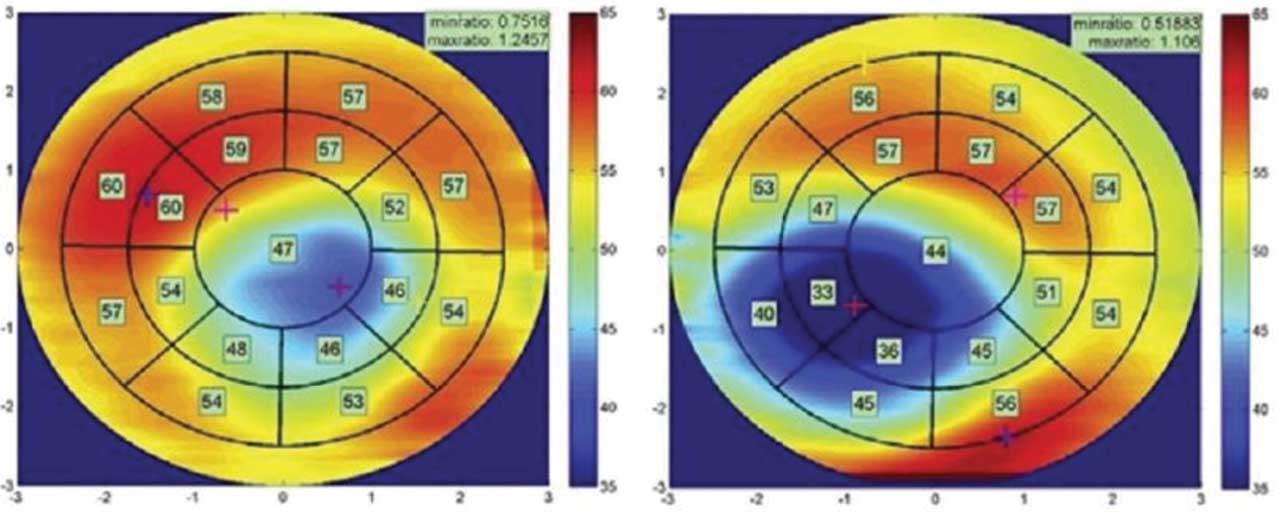
OPTICAL ABERROMETRY
Keratoconic corneas can cause optical aberrations when light transmits through their ectatic surface. In particular, higher-order aberrations (HOAs) from the anterior corneal surface, as measured by a wavefront aberrometer, can differentiate early, subclinical keratoconus from normal.4 HOA metrics, such as vertical coma or the root mean square error of horizontal and vertical coma, have shown particularly high sensitivities for detecting early or forme fruste keratoconus.9,10 Sensitivity further improves if anterior HOA metrics are combined with total wavefront HOA metrics or pachymetry;10 however, it does not significantly improve when posterior corneal HOA metrics are also considered.9
CORNEAL BIOMECHANICS
In addition to changes in the refractive qualities of the cornea, keratoconus affects its structural integrity. Recently, there has been significant interest in measuring in-vivo corneal biomechanical properties, such as elasticity and hysteresis, for detection of early keratoconus. The advent of new instruments that analyze deformation responses to measure these characteristics (eg, Ocular Response Analyzer, Reichert; CorVis ST, Oculus) may hold promise (Figure 3).11,12 However, there is limited evidence to support use of these tools for standalone screening.13 Rather, these instruments may provide additional measurements to help increase the sensitivity of more conventional modalities or may be useful in multivariate models of disease.4
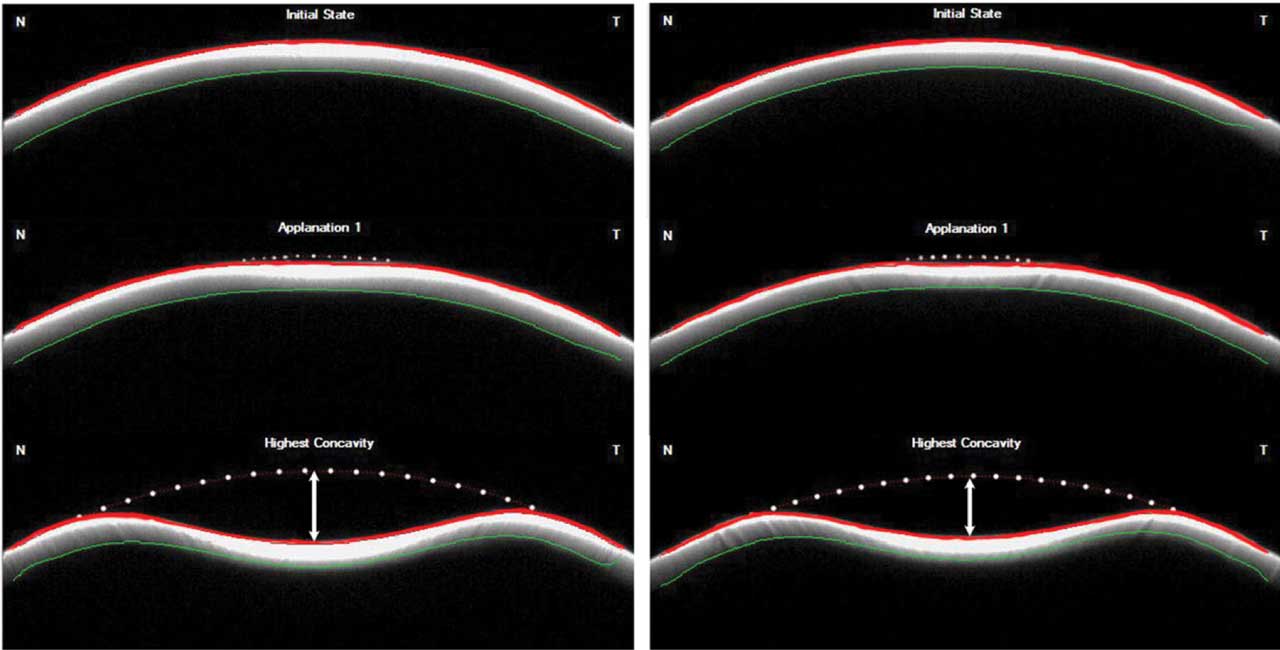
Further investigation is also needed on emerging technologies such as OCT-based elastography.
ARTIFICIAL INTELLIGENCE
The advent of artificial intelligence also holds promise for early detection of subclinical or forme fruste keratoconus. Multiple machine and deep learning algorithms have been developed based on a wide number of corneal variables with >95% sensitivity and specificity.14 KeratoDetect, is one such algorithm.15 By analyzing corneal topography images via a convolutional neural network (CNN), KeratoDetect can learn the unique features of a keratoconic eye for diagnostic use. It has a demonstrated accuracy of 99.3% at distinguishing keratoconic corneas from normal controls.15 Further development and application of these systems is currently of significant research interest.
21st CENTURY MODALITIES BRING HOPE
Based on global adoption of more advanced imaging modalities, we now know that keratoconus is more common than previously estimated and that its prevalence varies significantly with geographic location. While clinical and instrument-based diagnostics for manifest keratoconus have existed throughout the 20th century, our understanding of the natural history of keratoconus has improved significantly since that time. Modern-day modalities used for diagnosis, the most common of which are digital topography and tomography, are aimed at uncovering the earliest evidence of ectasia so that steps can be taken to halt progression, preserve vision and reduce the need for invasive surgery. Such screening tools continue to evolve, as does our therapeutic arsenal, making the future of keratoconus detection and management a bright one. OM
As for stopping progression …
The evolving array of screening tools for keratoconus has facilitated earlier detection over the last decade and improved long-term prognosis. Following a positive screening, if evidence of progressive disease is found, tertiary prevention strategies such as corneal crosslinking can decrease the need for future invasive corneal surgery.
The current standard for crosslinking is based on the Dresden protocol: Riboflavin solution is instilled on a corneal surface debrided of epithelium (“epi-off”) and irradiated with ultraviolet-A (UVA) light for 30 minutes.16 A safe and effective accelerated crosslinking protocol using more intense UV light has also been demonstrated;16 however, further randomized control trials are needed to confirm its safety and effectiveness over time. Risks of epi-off crosslinking include pain, reactivation of viral keratitis and corneal scarring, prompting efforts to develop a transepithelial protocol that doesn’t require epithelial debridement (“epi-on”).3
One technique for improving the efficacy of epi-on crosslinking is iontophoresis to enhance riboflavin absorption prior to corneal irradiation.17 While data on the effectiveness of epi-on crosslinking compared to epi-off is mixed,3 a recent multicenter randomized control trial comparing the protocols suggested superiority of epi-off approaches for the treatment of pediatric keratoconus.17,18
Aside from traditional crosslinking, emerging therapeutics include copper sulfate eyedrops (IVMED-80, Glaukos).3 Copper sulfate is a necessary cofactor for lysyl oxidase, the enzyme responsible for physiologic crosslinking of collagen molecules.3 Exogenously upregulating the function of lysyl oxidase therefore has the potential to stabilize corneal integrity. Early results from rabbit and human trials have been favorable;19 however, further studies are needed to ascertain long-term safety and efficacy.
While early crosslinking has the benefit of decreasing lifetime medical costs related to keratoconus, and improving quality of life,20 the out-of-pocket cost for the procedure can be significant for the uninsured or for patients not eligible for reimbursement. Additionally, socioeconomic factors may make it impractical for certain patient populations to attend a medical center that provides this procedure.
Further, at present there is only one FDA-approved crosslinking system in the United States — Glaukos’s iLink — which is only available at select locations. So, there remains a need for more cost-effective and accessible treatment options.
Preliminary evidence exists that one viable alternative may be high-dose oral riboflavin activated by ambient sunlight.21 In one study, three patients were able to achieve similar degrees of corneal flattening using this technique compared to conventional protocols.21 Although further research is needed, this method may prove to be a less expensive and more accessible, albeit slower, alternative to traditional crosslinking procedures.
Interest also surrounds exosomes as potential therapeutic delivery systems, though studies on safety and cost are limited. Exosomes are small, membrane-bound, extracellular vesicles secreted by cells that contain biomolecules capable of mediating cell-cell communication and tissue repair. Early results show that exosomes from multipotent corneal cell lines may be capable of inducing regeneration and reorganization of corneal tissue affected by keratoconus.3
References
- Bui AD, Truong A, Pasricha ND, Indaram M. Keratoconus Diagnosis and Treatment: Recent Advances and Future Directions. Clin Ophthalmol. 2023;17:2705-2718.
- Kanellopoulos AJ. Long term results of a prospective randomized bilateral eye comparison trial of higher fluence, shorter duration ultraviolet A radiation, and riboflavin collagen cross linking for progressive keratoconus. Clin Ophthalmol. 2012;6:97-101.
- Iqbal M, Gad A, Kotb A, Abdelhalim M. Analysis of the outcomes of three different cross-linking protocols for treatment of paediatric keratoconus: A multicentre randomized controlled trial. Acta Ophthalmol. 2024;102(1):e105-e116.
- Iqbal M, Elmassry A, Saad H, et al. Standard cross-linking protocol versus accelerated and transepithelial cross-linking protocols for treatment of paediatric keratoconus: a 2-year comparative study. Acta Ophthalmol. 2020;98(3):e352-e362.
- Molokhia S, Muddana SK, Hauritz H, et al. IVMED 80 eye drops for treatment of keratoconus in patients - Phase 1/2a. Investigative Ophthalmology & Visual Science. 2020;61:2587.
- Lindstrom RL, Berdahl JP, Donnenfeld ED, et al. Corneal cross-linking versus conventional management for keratoconus: a lifetime economic model. Journal of Medical Economics. 2021;24(1):410-420.
- Schaeffer, Karen, Jarstad, John, Schaeffer, Alan, McDaniel, Lindsay. Topographic corneal changes induced by oral fiboflavin in the treatment of corneal ectasia. Investigative Ophthalmology & Visual Science. 2018;59:1413.
- Torres Netto EA, Al-Otaibi WM, Hafezi NL, et al. Prevalence of keratoconus in paediatric patients in Riyadh, Saudi Arabia. Br J Ophthalmol. 2018;102:1436-1441.
- Gorskova EN, Sevost’ianov EN. [Epidemiology of keratoconus in the Urals]. Vestn Oftalmol. 1998;114:38-40.
- Santodomingo-Rubido J, Carracedo G, Suzaki A, Villa-Collar C, Vincent SJ, Wolffsohn JS. Keratoconus: An updated review. Cont Lens Anterior Eye. 2022;45:101559.
- Saad A, Gatinel D. Topographic and tomographic properties of forme fruste keratoconus corneas. Invest Ophthalmol Vis Sci. 2010;51:5546-5555.
- Schlegel Z, Hoang-Xuan T, Gatinel D. Comparison of and correlation between anterior and posterior corneal elevation maps in normal eyes and keratoconus-suspect eyes. J Cataract Refract Surg. 2008;34:789-795.
- Gomes JAP, Tan D, Rapuano CJ, et al. Global consensus on keratoconus and ectatic diseases. Cornea. 2015;34:359-369.
- Li Y, Chamberlain W, Tan O, Brass R, Weiss JL, Huang D. Subclinical keratoconus detection by pattern analysis of corneal and epithelial thickness maps with optical coherence tomography. J Cataract Refract Surg. 2016;42:284-295.
- Bühren J, Kook D, Yoon G, Kohnen T. Detection of subclinical keratoconus by using corneal anterior and posterior surface aberrations and thickness spatial profiles. Invest Ophthalmol Vis Sci. 2010;51:3424-3432.
- Bühren J, Kühne C, Kohnen T. Defining subclinical keratoconus using corneal first-surface higher-order aberrations. Am J Ophthalmol. 2007;143:381-389.
- Vellara HR, Patel DV. Biomechanical properties of the keratoconic cornea: a review. Clin Exp Optom. 2015;98:31-38.
- Masiwa LE, Moodley V. A review of corneal imaging methods for the early diagnosis of pre-clinical Keratoconus. J Optom. 2020;13:269-275.
- Steinberg J, Katz T, Lücke K, Frings A, Druchkiv V, Linke SJ. Screening for Keratoconus With New Dynamic Biomechanical In Vivo Scheimpflug Analyses. Cornea. 2015;34:1404.
- Cao K, Verspoor K, Sahebjada S, Baird PN. Evaluating the Performance of Various Machine Learning Algorithms to Detect Subclinical Keratoconus. Transl Vis Sci Technol. 2020;9:24.
- Lavric A, Valentin P. KeratoDetect: Keratoconus Detection Algorithm Using Convolutional Neural Networks. Comput Intell Neurosci. 2019;2019:8162567.