It is hard to believe the first successful cornea transplant was performed in 1905 considering the most successful solid organ transplant, the kidney, was not successfully performed until December 1954.1 The new millennium has seen an explosion of advancements for patients in need of cornea transplants to restore vision. What follows is a review of some of the most exciting surgical advances that will help us reach our goal of eradicating corneal blindness.
GAME CHANGER: ENDOTHELIAL KERATOPLASTY
The most groundbreaking advancement with the widest global impact for patients has been endothelial keratoplasty (EK), which has changed the standard of care for the surgical treatment of endothelial corneal disease. Endothelial corneal diseases include Fuchs’ endothelial corneal dystrophy, pseudophakic or aphakic bullous keratopathy, prior transplant rejection or failure, endothelial decompensation from infection or trauma, and more rare conditions such as congenital hereditary endothelial dystrophy, iridocorneal endothelial syndrome or posterior polymorphous corneal dystrophy.
The Eye Bank Association of America annual statistical report shows that since the inception of collecting numbers on EK surgeries in 2005, 2022 saw the highest number of EK procedures ever performed annually in the United States with 30,812 surgeries (Table).2 EK has become the standard of care for surgery in these patients in a relatively short amount of time (Figure 1).3,4
DOMESTIC SURGERY USE | 2013 | 2014 | 2015 | 2016 | 2017 | 2018 | 2019 | 2020 | 2021 | 2022 |
Penetrating Keratoplasty | 20,954 | 19,294 | 19,160 | 18,579 | 18,346 | 17,347 | 17,409 | 15,402 | 16,269 | 15,835 |
Endothelial Keratoplasty | 24,987 | 25,965 | 27,208 | 28,327 | 28,993 | 30,336 | 30,650 | 26,095 | 30,098 | 30,812 |
Anterior Lamellar Keratoplasty | 951 | 914 | 1.115 | 1,232 | 1,027 | 884 | 745 | 505 | 544 | 476 |
Keratolimbal Allograft | 91 | 80 | 97 | 82 | 93 | 68 | 95 | 109 | 124 | 107 |
K-Pro | 223 | 260 | 323 | 279 | 304 | 225 | 251 | 161 | 167 | 122 |
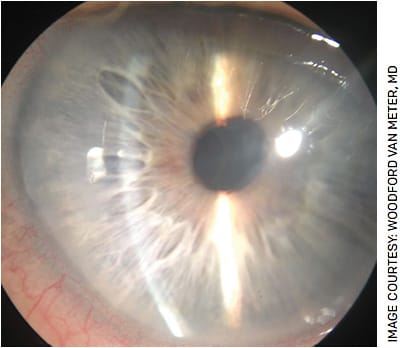
The ability to avoid penetrating keratoplasty (PK) for these patients has revolutionized their postoperative outcomes and severely limited the many sight-threatening complications that were possible with PK. Whether using the technique of Descemet stripping endothelial keratoplasty or Descemet membrane endothelial keratoplasty, studies show patients have quicker vision recovery, better long-term visual outcomes, less surgically induced astigmatism and fewer intraoperative and postoperative complications.3,4
FEMTOSECOND LASERS BRING NEW DEVELOPMENTS
Tissue preparation and technique
The advent of femtosecond lasers has led to the development of exciting advancements in tissue preparation and actually altered the keratoplasty technique. The femtosecond laser can be used by the eye bank for donor tissue preparation in both penetrating and anterior lamellar keratoplasty. The eye bank can fashion the diameter and type of side cut to perfect the donor tissue delivered to the surgeon.
From the surgeon’s perspective, utilizing femtosecond keratoplasty involves using it as the trephination device to cut the diseased cornea. Various patterns may be placed in the laser device, such as a mushroom or top-hat configuration or a zigzag cut, to ensure the proper fitting of donor and recipient at the graft-host junction. These cuts can be fashioned to match what was done on the donor tissue with slight oversizing of the donor cut done elsewhere. The femtosecond laser has also been used for preparation of donor tissue in EK surgery as a tool to provide the posterior donor lenticule prior to stripping of the host’s endothelium.5
Femto challenges and benefits
A major challenge of routine femtosecond use remains the transporting of the patient from the femtosecond laser to the operating microscope, depending on which technology is used. Some technologies have afforded the ability to do both in the same operating room. Also, the finances of using the femtosecond laser remain a challenge given insurers do not allow balanced billing to cover the cost of using the femtosecond laser interface for both the donor and recipient.
While deterrents exist to the technology, studies have found the interfaces after use of the laser provides better wound integrity, improved tectonic support and the ability to remove sutures earlier, affording quicker vision recovery.6
ARTIFICIAL CORNEAS
The development of an artificial cornea transplant remains a major area of research in preventing global corneal blindness in countries where donor cornea tissue is not a viable option. The only FDA-approved device for artificial cornea transplantation in the United States remains the Boston keratoprosthesis (KPro).
Early concerns with high rates of infection, device extrusion and loss of vision from glaucoma or retrocorneal membranes have dramatically decreased with the advancements by its innovator, Claes Dohlman, MD, PhD, and his research team. Major developments have included the addition of a titanium back plate with an increase in back plate apertures to provide the donor tissue carrier with nutrients and decrease rates of corneal melting. Long-term antibiotic prophylaxis and use of a therapeutic contact lenses have dramatically decreased infection risk and corneal melting as well. These advancements have afforded a large uptick in its use over the last few decades (Figure 2).
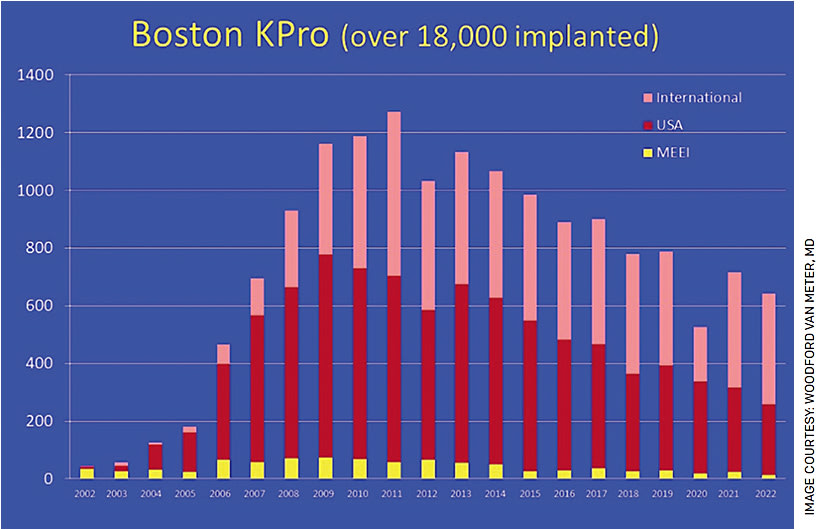
Surgical adjuncts to help with glaucoma treatment, such as glaucoma drainage devices, have also led to longer vision retention in these patients. As new techniques develop to lower the risk of vision loss from glaucoma, the procedure may yet prove to be a useful adjunct in the United States and across the world in fighting corneal blindness for a long time to come.7
New devices such as KeraMed’s KeraKlear artificial cornea, Gore’s synthetic cornea and CorNeat Vision’s CorNeat KPro device continue to be used on an experimental basis and have not seen FDA approval at this point.
CULTURED ENDOTHELIAL CELLS
Replacement for corneal tissue
The newest advancements in surgical treatment of corneal disease involves the delivery of cultured endothelial cells (CEC) as a replacement to using corneal tissue. A number of companies are making a push to start clinical trials in the United States in search of FDA approval to use CEC for corneal disease treatment.
The idea of using CEC as an alternative to EK in the replacement of a diseased corneal endothelium was conceptualized in 1978.8 A landmark publication by Kinoshita et al in 2018 showed restoration of corneal transparency in 11 of 11 treated eyes with endothelial corneal disease (100%; 95% confidence interval [CI], 72 to 100).7 CEC density ranged from 947 to 2833 cells per square millimeter in the 11 eyes (mean density, 1924 cells per square millimeter [95% CI, 1537 to 2312]).
How CEC works
Currently, isolation of CEC involves a two-step technique in which the Descemet membrane-endothelial layer is peeled from the cadaveric donor cornea as with EK and subjected to enzymatic digestion using collagenase, dispase and trypsin/EDTA. After isolation of CEC, the line undergoes cultivation and expansion within a culture medium. A large variety of growth-stimulating environments have been developed to support this cellular expansion. These specialized culture media together with various growth factors are shown to support CEC growth and proliferation. The growth factors enhance the CEC’s ability for expansion.
Additional additives to the culture medium include inhibitors to the p160-Rho-associated coiled-coil kinase (ROCK) signaling pathway.9 The pathway is suspected to lead to cell senescence. Blockage of the pathway with a ROCK inhibitor has been shown to promote the attachment and proliferation of CECs and affords improved survivability of the CEC and continued expansion of the culture line.9
Rho-associated protein kinase inhibitors have been shown to demonstrate stimulatory effects on endothelial cell proliferation, adherence and function. They also inhibit apoptosis and enhance post-wound cellular recovery.9
Once CECs have been successfully cultured in vitro, they are delivered to the posterior corneal surface of the recipient, adhere to the Descemet membrane/stroma and regulate corneal hydration to promote corneal clearing and transparency. This step can occur through cell injection intracamerally, on an ultra-thin monolayered sheet or via a cell-carrier system. Direct cell injection requires the patient to lie prone to allow gravity’s assistance in cell adherence.
Additionally, ferromagnetic induction or enhancements with substrates, such as ROCK inhibitor, may be used to promote adherence. Ultra-thin monolayers may be cultured on specially designed surfaces and mobilized upon the adjustment of the surface characteristics to deliver the CECs.
Aiming for the US market
Aurion Biotech and OcuCell are using proprietary technologies to obtain CEC and plan to deliver these tools to the surgical setting in the near future. The proprietary additives or enhancements to cultivate and expand CEC in the laboratory are what differentiate companies from one another. Additionally, EmmeCell is using ferromagnetic modified endothelial cells that are cultured, expanded and altered in the laboratory with proprietary techniques. After the cells are delivered, a special magnetized contact lens is worn to hold the CEC against the recipient stroma for a short period.
The challenges for CEC
For CEC tissue engineering to hit primetime, several obstacles must be overcome. First, a reproducible and mainstream protocol must be developed. Secondly, funding must be achieved to overcome the expense to cultivate and expand CEC in the laboratory. Lastly, if FDA approval is achieved, patients must be able to afford these expensive technologies and insurance companies must get on board for coverage to make the procedure a mainstream option.
CONCLUSION
While innovations in corneal surgery have exploded in the new millennium, advancements in EK will continue to be the mainstay of surgical treatment. Despite the success of EK, tissue-engineered endothelial cells has the chance to become more mainstream in the near future. Three of the companies working on cultured endothelial cells begin Phase 3 studies this year for possible submission to the FDA. New artificial cornea devices are also close to submission for FDA review for eyes with past cornea transplants.
It is truly an exciting era for cornea surgeons as we will see amazing innovations over the next 5 years that will likely change our care and help with the potential eradication of corneal blindness for the future. OM
REFERENCES
- Mannis MJ, Mannis AA. Corneal Transplantation: A History in Profiles. 1999, J P Wayenborgh.
- Eye Bank Association of America. 2022 eye banking statistical report. https://restoresight.org/members/publications/statistical-report . Accessed May 1, 2023.
- Lee WB, Jacobs DS, Musch DC, et al. Descemet’s Stripping Endothelial Keratoplasty: Safety and Outcomes. Ophthalmology 2009;116:1818-1830.
- Deng SX, Lee WB, Hammersmith KM, et al. DMEK: Safety and outcomes: A report by the AAO. Ophthalmology. 2018;125:295-310.
- Jardine GJ, Holiman JD, Galloway JD, et al. Eye bank-prepared femtosecond laser-assisted automated Descemet Membrane endothelial grafts. Cornea. 2015;34:838-843.
- Wade M, Muniz Castro H, Garg S, et al. Long-term results of femtosecond laser-enabled keratoplasty. Cornea 2019:38;42-49.
- Lee WB, Shtein RM, Kaufman SC, et al. Boston keratoprosthesis: Outcomes and complications. Ophthalmology. 2015:122;1504-1511.
- Kinoshita S, Koizumi N, Ueno M, et al. Injection of cultured cells with a ROCK inhibitor for bullous keratopathy. NEJM. 2018;378:995-1003.
- Okumura N, Ueno M, Koizumi N, et al. Enhancement on primate corneal endothelial cell survival in vitro by a ROCK inhibitor. Invest Ophthalmol Vis Sci. 2009;50;3680.