TAKE-HOME POINTS
- AAVs and coadministration with oral steroids have lowered the risk of immunologic events compared to early gene therapy studies.
- There are a large number of actively recruiting gene therapy clinical trials for various inherited and non-inherited common retinal disease.
- The newest mode of gene therapy delivery is via suprachoroidal injection.
- Breakthroughs in gene therapy continue to be occur. Many of the diseases were previously thought to have no treatment, and even halting progression can be considered a success.
Thanks to some promising trends in gene therapy for both inherited and acquired retinal diseases, previously “nontreatable” retinal diseases now have a potential avenue for successful treatment.
We have seen a lot of momentum since the first gene therapy for retina was approved by the FDA in 2017, with several exciting new trials.
To understand these latest updates, it is important to have an understanding of the science behind gene therapy in retina. Gene therapy involves altering genetic material to either increase production of a protein or delete genetic material responsible for proteins that are causing issues. At this time, the majority of the gene therapy trials utilize the concept of introducing modified genetic material to change protein expression,1 but some emerging trials are utilizing CRISPR/Cas9 gene-editing technologies (CRISPR Therapeutics) to delete genetic material or RNA-based gene therapy, which utilizes RNA molecules to alter production of proteins.
In this article, we’ll discuss the advantages and disadvantages of some vectors, modes of gene therapy delivery and mutation dependent/independent approaches.
SELECTING A VECTOR
Different vectors have their advantages and disadvantages. The ideal vector is one that has a high efficacy, a long-lasting effect and a low rate of immunogenicity. Commonly used vectors include adenovirus, adeno-associated adenovirus, lentivirus and non-viral vectors.
Adenovirus vector
Adenovirus was the first vector utilized in clinical trials, but it is rarely used now due to its propensity to cause severe immune responses.2,3 Instead, adeno-associated virus (AAV) is now the most commonly used vector in gene therapy trials. AAV is a small virus that is known for its relatively lower immunogenicity and its relatively high effectiveness at transducing nearby cells.4 However, its small size limits its ability to deliver larger genes.
Lentivirus vector
Lentivirus is a larger vector that was considered useful for delivering larger genes. However, lentiviruses are thought to be more immunogenic than AAVs.5
Non-viral vector
Non-viral vectors have been attempted as well, including lipid-based systems, polymers and peptides that form nanocomplexes. While these are less immunogenic, they do not have as good efficacy in gene transduction and are not as widely used.6
MODES OF DELIVERY
Subretinal injections
Subretinal delivery is the most common method of delivering gene therapy. Subretinal injections have existed for decades7 and are ideal in gene therapy because they deliver the vector directly adjacent to the photoreceptors and retinal pigment epithelium (RPE), which are commonly dysfunctional in inherited retinal diseases. The technique involves first performing a complete vitrectomy then utilizing a 41-G cannula to access the subretinal space through the retina (Figure). While this is an advanced surgical technique, many retina surgeons should be able to perform it after some training.
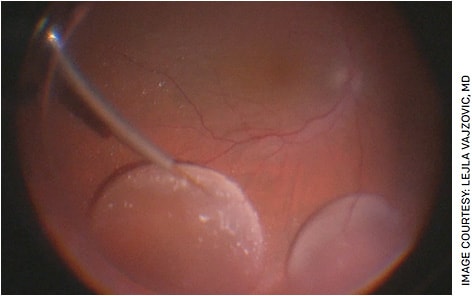
Disease | Gene | Delivery Method | Clinical Trial ID | Name | Vector/Mechanism | Company/Sponsor |
---|---|---|---|---|---|---|
Leber’s congenital amaurosis | RPE65 | Subretinal | FDA approved | Voretigene neparvovec-rzyl (Luxturna) | AAV | Spark Therapeutics |
CEP290 | Subretinal | NCT03872479 | EDIT-101 | Using CRISPR/Cas9 | Editas Medicine | |
CEP290 | Intravitreal | NCT03913143 | QR-110 (Sepofarsen) | RNA antisense oligonucleotides | ProQR Therapeutics | |
Retinitis pigmentosa | RPGR | Subretinal | NCT03252847NCT04671433 | botaretigene sparoparvovec (formerly AAV-RPGR) | AAV | MeiraGTx/Janssen Pharmaceuticals |
RPGR | Subretinal | NCT04850118 | AGTC-501 | AAV | Applied Genetic Technologies Corp (AGTC) | |
Gene-independent | Intravitreal | NCT03326336 (PIONEER) | GS030-DP | AAV/Optogenetics | GenSight Biologics | |
Gene-independent | Intravitreal | NCT04945772 (RESTORE) | MCO-010 | AAV/Optogenetics | Nanoscope Therapeutics | |
X-linked juvenile retinoschisis | RS1 | Intravitreal | NCT02317887 | AAV8-scRS/IRBPhRS | AAV | NEI |
RS1 | Intravitreal | NCT0241662 | rAAV2tYF-CB-hRS1 | AAV | AGTC | |
Choroideremia | CHM | Subretinal | NCT01461213 NCT02077361 NCT02553135 NCT02671539 NCT02341807 NCT02407678 (REGENERATE TRIAL) NCT03507686 (GEMINI TRIAL) NCT03496012 (STAR TRIAL) NCT04483440 |
AAV2-REP1 | AAV | University of Oxford, University of Alberta, University of Miami, STZ eyetrial, Spark Therapeutics, Nightstar Therapeutics, 4D Molecular Therapeutics |
Achromatopsia | CNGA3 | Subretinal | NCT02935517 | AAV2tYF-PR1.7-hCNGA3 | AAV | AGTC |
CNGB3 | Subretinal | NCT02599922 | AAV2tYF-PR1.7-hCNGB3 | AAV | AGTC | |
CNGB3 | Subretinal | NCT03001310 | AAV8-hCAR-hCNGB3 (Entacingene turiparvovec) | AAV | MeiraGTx/Janssen | |
CNGA3 | Subretinal | NCT03758404 | AAV8-hG1.7-hCNGA3 | AAV | MeiraGTx/Janssen | |
CNGA3 | Subretinal | NCT03758404 | AAV8-hG1.7-hCNGA3 | AAV | MeiraGTx/Janssen | |
Stargardt disease | ABCA4 | Subretinal | NCT01367444 | SAR422459 | Lentiviral | Sanofi |
Wet Macular Degeneration | ABCA4, ELOVL4, or PROM 1 | Intravitreal | NCT05417126 (STARLIGHT) | MCO-010 | AAO/Optogenetics | Nanoscope Therapeutics |
Gene-independent | Intravitreal | NCT05197270 (PRISM) | 4D-150 | AAV | 4DMT | |
Gene-independent | Subretinal | NCT04704921 (ATMOSPHERE) NCT05407636 (ASCENT) |
ABBV-RGX-314 | AAV | Regenxbio | |
Gene-independent | Suprachoroidal | NCT04514653 | RGX-314 | AAV | Regenxbio |
Intravitreal injections
Intravitreal injections have also been utilized for gene therapy. However, they are thought to target mostly the inner retina with less reach to the photoreceptor and RPE cells, which limits its utility in many retinal diseases.8 Some studies suggest that intravitreal injections can mount a greater immune response than subretinal injections and that the ILM limits diffusion of the AVV to the retina.9,10 New technologies using directed evolution have been developed to bypass this barrier, but intravitreal delivery route is still in earlier stages of investigation compared to subretinal injection route.11
Suprachoroidal injections
Recently, suprachoroidal delivery has been explored as a means of delivering the vector to the retina. This delivers the medication into the potential space between the choroid and the sclera, hypothetically having an effect on the RPE and outer retina while avoiding a surgical procedure necessary for subretinal gene delivery and avoiding vector exposure to the anterior segment compared to intravitreal delivery. Studies have shown that suprachoroidal injections have a similar efficacy as subretinal injections for gene therapy.12
MUTATION DEPENDENT THERAPIES
Leber congenital amaurosis
Voretigene neparvovec-rzyl (Luxturna, Spark Therapeutics) is the first and only FDA-approved gene therapy for biallelic mutations of RPE65. RPE65 encodes for an enzyme necessary for converting 11-trans retinyl to 11-cis-retinol, which is a key part of the photoreceptor visual cycle.13 Lack of this enzyme leads to severe and progressive vision loss from childhood. Diseases that are biallelic mutations of RPE65 include autosomal dominant Leber congenital amaurosis (LCA) and retinitis pigmentosa (RP). In order to be eligible, a genetic test must confirm the biallelic mutations in RPE65.
In the Phase 3 clinical trial for Luxturna, patient outcomes included a standardized multi-luminance mobility testing (which was a functional measure of navigating through a specifically designed obstacle course), full-field light sensitivity threshold testing and visual acuity. Patients who received Luxturna were significantly more likely to pass the multiluminance mobility testing and have a lower full-field light sensitivity threshold, but there was no significant difference in visual acuity.
It is also important to understand the side effects of Luxturna and gene therapy. Two adverse events noted in the Phase 3 trial included foveal thinning and a sustained reduction in visual acuity.14 Luxturna is an AAV delivered via subretinal injection, and it is co-administered with oral steroids to suppress some of the immunogenic reaction.
CRISPR/Cas9 technology (CRISPR Therapeutics) is also being used in the treatment of LCA by removing a point mutation in the CEP290 gene in a trial led by Editas Medicine.15
ProQR Therapeutics is also utilizing RNA antisense oligonucleotides to prevent abnormal splicing of the CEP290 protein, allowing for normal production. This Phase 1/2 clinical trial has published results and demonstrated improvement in visual acuity in a post-hoc analysis and relatively manageable safety profile, though they did not meet their primary endpoint.16
X-linked RP
X-linked RP is another early and progressive cause of vision loss in children. It is associated with many genes, including RPGR, which causes one of the most severe forms of RP.17,18 Multiple gene therapy trials are ongoing including one run by MeiraGTx and Janssen Pharmaceuticals (pharmaceutical companies of Johnson & Johnson) for an AAV vector delivered subretinally; it has been studied in Phase 1/2 dose escalation trials (MGT009), and Phase 3 is currently ongoing (NCT04671433).
Similarly, Applied Genetic Technologies Corp (AGTC) has another AAV vector delivered subretinally that is in an ongoing Phase 2/3 trial.
X-linked juvenile retinoschisis
X-linked juvenile retinoschisis (XLRS) is commonly caused by a mutation in the retinoschisin 1 gene, which encodes for a protein that helps with retinal organization and intercellular adhesion. Within this gene, patients can get fovea-splitting schisis. Due to the poor integrity of the retina, most of the gene therapy trials involving XLRS are delivered intravitreally and not subretinally. The thought is that patients with XLRS have a weakened ILM, which actually helps facilitate more diffusion of the vector to the retina.
Two trials are utilizing different AAV to deliver the gene intravitreally. One was a dose-escalation study that demonstrated structural improvement of cystic spaces compared to untreated eye without improvement of visual acuity. Overall, there was a dose-dependent inflammatory response noted, which was adequately treated with oral steroids.19
AGTC had a Phase 1/2 clinical trial that did not demonstrate clinical benefit after 6 months.20
Choroideremia
Choroideremia is associated with the CHM gene that encodes for a protein responsible for intracellular vesicular transport ras-associated binding excort protein 1 (REP1) — lack of which leads to death of RPE, photoreceptors and choroid. The small size of the CHM gene makes it a good candidate for gene therapy. There are multiple studies involving AAV2-REP1 in treatment of choroideremia. Phase 1 and 2 trials demonstrated reasonable safety with variable visual acuity outcomes,21 though the Phase 3 STAR trial did not meet the primary endpoint of at least 15 ETDRS letters of improvement from baseline.
Achromatopsia
Achromatopsia is a disease that affects all three types of cone photoreceptors, leading to poor visual acuity and significant difficulty distinguishing colors. Several Phase 1/2 trials that utilize AAV with genes CNGA3 and CNGB3 have been completed,22 the first of which had good safety outcomes at 1 and 3 years.23,24 There are several ongoing Phase 1/2 trials for achromatopsia.
Stargardt disease
The most common mutation leading to Stargardt disease is in the gene ABCA4, which results in lipofuscin accumulation in the RPE and ultimately leads to atrophy, degeneration and vision loss. There is a Phase 1/2 clinical trial utilizing lentivirus to delivered ABCA4 that was terminated in 2015 due to lack of sponsorship.25 However, Sanofi just published their 3-year data on safety, which was overall positive.26
MUTATION-INDEPENDENT THERAPIES
Optogenetics
Optogenetics utilizes a mutation-independent approach, introducing genes that produce general opsins with the goal of improving visual function even without functioning photoreceptors.27 These opsins directly target downstream bipolar and retinal ganglion cells and therefore do not require functioning or even existing photoreceptors to work. Four clinical trials are ongoing studying the use of optogenetics in RP. These typically introduce AAV vectors through intravitreal injections.
Nanoscope Therapeutic’s trial for MCO-010 recently demonstrated good safety profile with improvement in visual function. Optogenetic technology is also being used in Stargardt disease.
AMD, diabetic retinopathy and diabetic macular edema
Similar to optogenetics, there has been a recent push to use gene therapy to create ocular “bio-factory” with long-term anti-VEGF production. Adverum Biotechnologies utilized intravitreal injection of an AAV.7M8 vector in diabetic macular edema in the INFINITY study, but the trial was stopped early for adverse events of choroidal effusion and hypotony in the high dose group. Adverum’s neovascular AMD trial, OPTIC, with intravitreal injection approach did not have the same safety concerns as the INFINITY study.28
4DMT has single-dose intravitreal AAV vector carrying transgenes encoding for aflibercept and an miRNA sequence targeting VEGF-C for treatment of neovascular AMD. Khanani et al presented interim data from the Phase 1/2 clinical trial, PRISM, demonstrating that these injections were safe and well tolerated, and reduced treatment burden.
Regenxbio has had positive Phase 1b/2a subretinal delivery study with excellent efficacy and safety profiles and is currently recruiting for two pivotal trials: ATMOSPHERE and ASCENT. Regenxbio is also running several trials that utilize suprachoroidal injections of the AAV vector for patients with neovascular AMD (AVVIATE trial) and diabetic retinopathy without macular edema (ALTITUDE trial) and early results are promising.
CONCLUSION
This is an exciting time for gene therapy in retina. Gene therapy has the potential to transform how we think about many of these diseases that previously did not have any treatment. OM
REFERENCES
- Dhurandhar D, Sahoo NK, Mariappan I, Narayanan R. Gene therapy in retinal diseases: A review. Indian J Ophthalmol. 2021;69(9):2257-2265.
- Raper SE, Chirmule N, Lee FS, et al. Fatal systemic inflammatory response syndrome in a ornithine transcarbamylase deficient patient following adenoviral gene transfer. Mol Genet Metab. 2003;80(1-2):148-158.
- Ghoraba HH, Akhavanrezayat A, Karaca I, et al. Ocular Gene Therapy: A Literature Review with Special Focus on Immune and Inflammatory Responses. Clin Ophthalmol. 2022;16:1753-1771. Published June 3, 2022.
- Buck TM, Wijnholds J. Recombinant Adeno-Associated Viral Vectors (rAAV)-Vector Elements in Ocular Gene Therapy Clinical Trials and Transgene Expression and Bioactivity Assays. Int J Mol Sci. 2020;21(12):4197. Published June 12, 2020.
- Kalesnykas G, Kokki E, Alasaarela L, et al. Comparative Study of Adeno-associated Virus, Adenovirus, Bacu lovirus and Lentivirus Vectors for Gene Therapy of the Eyes. Curr Gene Ther. 2017;17(3):235-247.
- Bordet T, Behar-Cohen F. Ocular gene therapies in clinical practice: viral vectors and nonviral alternatives. Drug Discov Today. 2019;24(8):1685-1693.
- Irigoyen C, Amenabar Alonso A, Sanchez-Molina J, Rodríguez-Hidalgo M, Lara-López A, Ruiz-Ederra J. Subretinal Injection Techniques for Retinal Disease: A Review. J Clin Med. 2022;11(16):4717. Published Aug. 12, 2022.
- Planul A, Dalkara D. Vectors and Gene Delivery to the Retina. Annu Rev Vis Sci. 2017;3:121-140.
- Reichel FF, Peters T, Wilhelm B, et al. Humoral Immune Response After Intravitreal But Not After Subretinal AAV8 in Primates and Patients. Invest Ophthalmol Vis Sci. 2018;59(5):1910-1915.
- Dalkara D, Kolstad KD, Caporale N, et al. Inner limiting membrane barriers to AAV-mediated retinal transduction from the vitreous. Mol Ther. 2009;17(12):2096-2102.
- Dalkara D, Byrne LC, Klimczak RR, et al. In vivo-directed evolution of a new adeno-associated virus for therapeutic outer retinal gene delivery from the vitreous. Sci Transl Med. 2013;5(189):189ra76.
- Ding K, Shen J, Hafiz Z, et al. AAV8-vectored suprachoroidal gene transfer produces widespread ocular transgene expression. J Clin Invest. 2019;129(11):4901-4911. Published Aug. 13, 2019.
- Redmond TM, Poliakov E, Yu S, Tsai JY, Lu Z, Gentleman S. Mutation of key residues of RPE65 abolishes its enzymatic role as isomerohydrolase in the visual cycle. Proc Natl Acad Sci U S A. 2005;102(38):13658-13663.
- Russell S, Bennett J, Wellman JA, et al. Efficacy and safety of voretigene neparvovec (AAV2-hRPE65v2) in patients with RPE65-mediated inherited retinal dystrophy: a randomised, controlled, open-label, phase 3 trial [published correction appears in Lancet. Aug 26, 2017;390(10097):848]. Lancet. 2017;390(10097):849-860.
- First CRISPR therapy dosed. Nat Biotechnol. 2020;38(4):382.
- Russell SR, Drack AV, Cideciyan AV, et al. Intravitreal antisense oligonucleotide sepofarsen in Leber congenital amaurosis type 10: a phase 1b/2 trial. Nat Med. 2022;28(5):1014-1021.
- Hosch J, Lorenz B, Stieger K. RPGR: role in the photoreceptor cilium, human retinal disease, and gene therapy. Ophthalmic Genet. 2011;32(1):1-11.
- Nuzbrokh Y, Ragi SD, Tsang SH. Gene therapy for inherited retinal diseases. Ann Transl Med. 2021;9(15):1278.
- Cukras C, Wiley HE, Jeffrey BG, et al. Retinal AAV8-RS1 Gene Therapy for X-Linked Retinoschisis: Initial Findings from a Phase I/IIa Trial by Intravitreal Delivery. Mol Ther. 2018;26(9):2282-2294.
- Yang YP, Jheng YC, Chien Y, et al. Clinical manifestation and current therapeutics in X-juvenile retinoschisis. J Chin Med Assoc. 2022;85(3):276-278.
- Abbouda A, Avogaro F, Moosajee M, Vingolo EM. Update on Gene Therapy Clinical Trials for Choroideremia and Potential Experimental Therapies. Medicina (Kaunas). 2021;57(1):64. Published 2021 Jan 12.
- Michalakis S, Gerhardt M, Rudolph G, Priglinger S, Priglinger C. Achromatopsia: Genetics and Gene Therapy. Mol Diagn Ther. 2022;26(1):51-59.
- Fischer MD, Michalakis S, Wilhelm B, et al. Safety and Vision Outcomes of Subretinal Gene Therapy Targeting Cone Photoreceptors in Achromatopsia: A Nonrandomized Controlled Trial. JAMA Ophthalmol. 2020;138(6):643-651.
- Reichel FF, Michalakis S, Wilhelm B, et al. Three-year results of phase I retinal gene therapy trial for CNGA3-mutated achromatopsia: results of a non randomised controlled trial. Br J Ophthalmol. 2022;106(11):1567-1572.
- Parker MA, Choi D, Erker LR, et al. Test-Retest Variability of Functional and Structural Parameters in Patients with Stargardt Disease Participating in the SAR422459 Gene Therapy Trial. Transl Vis Sci Technol. 2016;5(5):10. Published Oct. 1, 2016
- Parker MA, Erker LR, Audo I, et al. Three-Year Safety Results of SAR422459 (EIAV-ABCA4) Gene Therapy in Patients With ABCA4-Associated Stargardt Disease: An Open-Label Dose-Escalation Phase I/IIa Clinical Trial, Cohorts 1-5. Am J Ophthalmol. 2022;240:285-301.
- Pan ZH, Lu Q, Bi A, Dizhoor AM, Abrams GW. Optogenetic Approaches to Restoring Vision. Annu Rev Vis Sci. 2015;1:185-210.
- Constable IJ, Pierce CM, Lai CM, et al. Phase 2a Randomized Clinical Trial: Safety and Post Hoc Analysis of Subretinal rAAV.sFLT-1 for Wet Age-related Macular Degeneration. EBioMedicine. 2016;14:168-175.