The introduction of optical coherence tomography (OCT) into clinical practice in the early 2000s revolutionized the way ophthalmologists visualize the structure of the retina and optic nerve. Until recently, limitations in OCT machine design and software prevented its use in retinal vasculature imaging. However, the development of machines with faster scanning speeds and new motion-detection algorithms paved the way for the next step in the evolution of OCT: optical coherence tomography angiography (OCTA).
OCTA allows ophthalmologists to obtain incredibly detailed, 3D images within seconds and without the use of invasive dyes. Though its limitations prevent it from replacing traditional imaging techniques such as fluorescein angiography (FA) and indocyanine green angiography (ICGA) at this time, OCTA may serve as a useful adjunct in diagnosing and monitoring several conditions like diabetic retinopathy, retinal vein occlusions and glaucoma.
We will take a closer look at OCTA and its value in treating challenging ophthalmic conditions.
FIRST, THERE WAS OCT
OCT imaging relies on the use of low-coherence interferometry — a technique in which a low-coherence light beam is shown onto a partially transparent sample (such as the retina), and the reflection of light against each layer of the sample is interpreted by an interferometric detector. The measurement of the sample’s reflectivity at many depths creates an “A-scan,” and several A-scans are obtained by passing the light-beam linearly across a sample to create a cross-section called a “B-scan.” Collecting several B-scans allows the algorithm to generate a three-dimensional cube scan and an “en face” image like one would obtain from traditional photography of the fundus. Early OCT machines, termed time-domain OCT, relied on a moving reference mirror, which reduces the scanning speed and therefore maximum resolution of the machine.1
These have since been phased out by more modern swept-source (SS-OCT) and source-domain (SD-OCT) machines that rely on a fixed mirror and fast-sweeping light beam to provide higher resolution images.1 While OCT imaging has provided unparalleled information regarding the structure of the various retinal layers, until recently it had been unable to visualize retinal vasculature due to technological limitations.
TRADITIONAL IMAGING OF RETINAL VASCULATURE
Traditionally, the gold standards of retinal vasculature imaging have been FA for retinal vessels and ICGA for the choroid.2,3 These techniques involve serial imaging of the fundus after intravascular injection of a fluorescent dye and offer several advantages. First, they capture wide-view, two-dimensional images of the fundus that allow visualization of the peripheral retinal vasculature. Secondly, by relying on serial imaging of a flowing dye, these techniques can provide information on the health of the retinal vasculature in addition to its structure by visualizing vessel leaks and filling times.3
Unfortunately, image acquisition is slow — the patient is imaged over 5-10 minutes. Additionally, because these images are two-dimensional, they are inherently limited in their ability to provide information about individual retinal layers. For example, superficial vessels can impair visualization of the deeper vasculature, and contrast extravasation in leaks can obscure the underlying retina. These techniques are also invasive, and the use of intravascular dyes carries its own associated adverse effects like gastrointestinal distress, skin reactions, headaches and even anaphylactic reactions in rare cases.2-4
NEXT GENERATION: OCT ANGIOGRAPHY
Advances in OCT imaging devices and software have led to the most recent development in retinal vascular imaging — OCTA, first introduced into clinical practice in 2015. OCTA algorithms measure the difference in light reflectivity caused by motion between subsequent B-scans. In a still eye, the primary source of motion is the flow of erythrocytes through retinal vessels, which allows for accurate mapping of the retinal vasculature.2-4 Perhaps the biggest advantage of OCTA is the unprecedented level of detail it can provide about the vasculature at various depths of the retina. For example, OCTA algorithms have automated segmentation features that allow users to visualize the superficial capillary plexus (SCP), deep capillary plexus (DCP) and choriocapillaris individually (Figure 1).2-4 In addition, acquisition time for OCTA images is on the order of seconds rather than minutes and does not require injection of dyes.
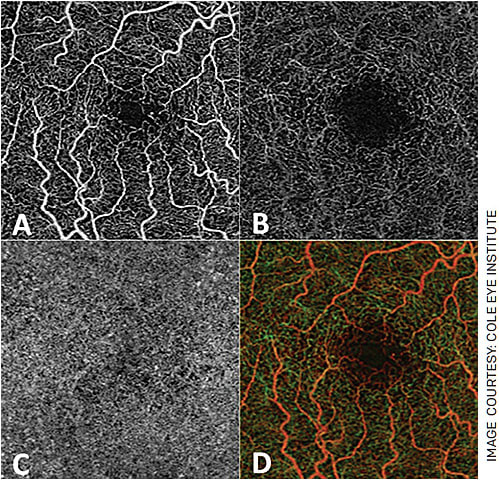
FIGURE 1. OCTA allows for visualization of the superficial capillary layer (A), deep capillary layer (B) and choriocapillaris (C) individually. It also produces an “en face” image of the retina in its entirety and can produce depth-encoded images (D), where superficial vessels appear as red-orange and deeper vessels appear green.
However, OCTA comes with its own limitations. The number of B-scans per image is fixed, regardless of the size of the imaging field. This leads to a significant drop-off in image resolution in larger scans, limiting the field of view for most commercially available OCTA devices to 10-30°.2 This is significantly less than FA and ICGA, which can provide up to a 200° field of view and include the peripheral retina.2 And, while OCTA can demonstrate the vascular network structure with fine detail, it is unable to capture dynamic processes like vessel leaks and filling times since it does not use a dye.2-4 Finally, because OCTA algorithms rely on detecting changes between subsequent B-scans secondary to motion, they are significantly more sensitive to motion artifacts than FA or ICGA.
AVAILABLE DEVICES
Several OCTA devices have been developed, each with their respective benefits and drawbacks. To date, the FDA has granted approval to:
- Zeiss Angioplex
- Zeiss PLEX Elite 9000
- Optovue Angiovue
- Topcon Triton
- Heidelberg Spectralis
Most commercially available OCTA machines employ SD technology. SD-OCT uses a broad-bandwidth light source at a shorter wavelength of around 840 nm, while SS-OCT employs a narrow-bandwidth light beam swept over a broad range of frequencies and with a longer wavelength around 1,000 nm.1 The longer wavelength used in SS-OCT reduces its axial resolution.1,4 The laser used is also significantly more expensive, and imaging is more prone to motion artifacts than in SD-OCT.
However, the narrow-bandwidth beam employed by SS-OCT allows for significantly deeper penetration into the eye without a drop-off in signal, allowing for enhanced visualization of deeper structures such as the choroid plexus.1 SS-OCT is also capable of higher scanning speeds and the ability to maintain resolution with larger image areas than SD-OCT.3
Each machine uses a unique image processing algorithm as well, and algorithms can broadly be divided into phase-based, amplitude-based and complex-signal-based methods.2 Most OCTA devices use an amplitude-based method; the first amplitude-based algorithm was termed split-spectrum amplitude decorrelation angiography, and it divides the OCT spectrum into bins to create several low-resolution scans that undergo decorrelation analysis and are then recombined to reduce noise.2,5 Optical microangiography is an example of a complex signal-based algorithm, which is more sensitive to low-velocity blood flow but also to motion artifacts.2
Other unique features include the concept of image averaging, which takes multiple OCTA scans and averages them to improve the signal-to-noise ratio. Additionally, several machines have built-in software that can quantify aspects of the retinal vasculature such as the foveal avascular zone (FAZ) size and vessel density.
APPLICATIONS IN CLINICAL PRACTICE
OCTA can detect abnormalities in the retinal vasculature associated with glaucoma and retinal diseases and has even been used for anterior segment imaging. In glaucoma, OCTA can detect attenuation of the peripapillary vascular network and decreased flow index around the optic nerve. This decreased flow index has high specificity and sensitivity for differentiating glaucomatous and non-glaucomatous eyes.3
In diabetes, OCTA can identify microaneurysms and is a good tool for detecting intraretinal microvascular abnormalities and differentiating them from neovascularization. It can detect early changes in diabetic eyes before clinical retinopathy develops, including increased FAZ size and irregularity and decreased vessel density (Figure 2).5,6
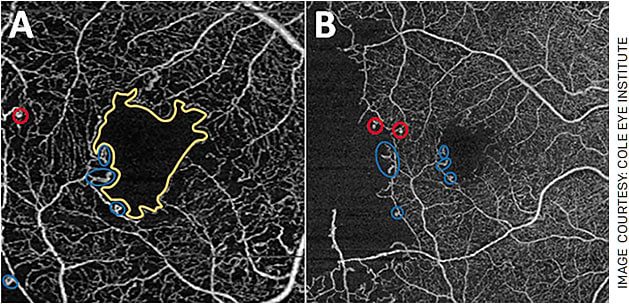
FIGURE 2. OCTA changes in diabetic retinopathy (DR). The figure contains (A) 3x3 mm and (B) 6x6 mm images of a patient’s right eye affected by proliferative DR that has been treated by pan-retinal laser photocoagulation. Note the increased size and irregularity of the FAZ (yellow outline), microaneurysms (red circle) and abnormal vessel loops (blue circles). In 2B, a large area of hypoperfusion can be seen as well.
In wet AMD, OCTA is an excellent tool for detecting choroidal neovascular membranes (CNVM) as well as classifying them. It can identify early Type I CNVMs that are not obvious on FA, potentially allowing for earlier treatment initiation, and can be used to monitor morphological changes in CNVMs during treatment with anti-VEGF injections.3,5
In retinal vein occlusions, OCTA may be as capable as FA in evaluating macular changes such as non-perfusion areas, vascular abnormalities like shunt vessels and FAZ changes when combined with OCT (Figure 3).5,7 In retinal artery occlusions, OCTA can quantify reduced capillary perfusion in the DCP and SCP and shows increased irregularity in the FAZ compared to healthy eyes.8 OCTA has also been effective in identifying CNVM in chronic central serous chorioretinopathy, characterizing vascular changes associated with macular telangiectasias and detecting changes in the SCP and DCP in uveitis.5,9
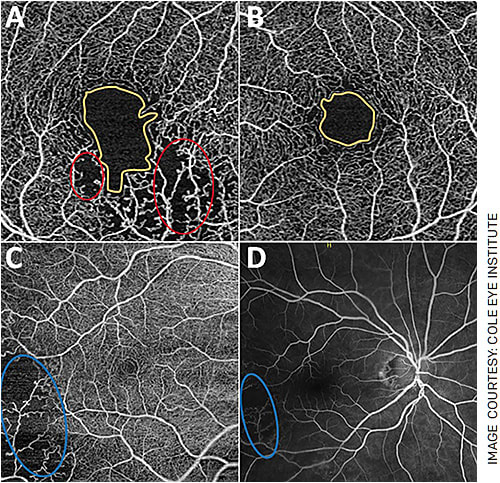
FIGURE 3. OCTA changes in branched retinal vein occlusions (BRVO). A: A patient’s right eye affected by a BRVO. B: The patient’s unaffected left eye. Note the increased size and irregularity of the FAZ in the affected eye (yellow outlines). FAZ irregularity has been quantified in the literature with a term called the “acircularity index,” which is increased in pathologies like BRVO and diabetic retinopathy. Also note areas of hypoperfusion (A) circled in red. C: The eye of another patient affected by BRVO. Note the large area of hypoperfusion circled in blue, which correlates well with the patient’s FA imaging (D).
BEWARE THESE COMMON PITFALLS
When analyzing OCTA images, it is critical to be aware of some of the common imaging artifacts you may encounter. Most commercial machines use automated segmentation algorithms to divide the retinal vascular layers. Segmentation artifacts occur when these automated algorithms incorrectly demarcate the layers and require manual adjustment by the ophthalmologist to correct. Shadowing artifacts can occur in eyes with corneal pathology, cataracts and other abnormalities, which can cause signal attenuation and generally appear as areas of decreased flow information.
Projection artifacts are common in all OCT devices (Figure 4) and result in projection of the images of superficial vessels onto the deeper vascular networks. Finally, because of the nature of OCTA, it is sensitive to motion and blinking artifacts that can cause image distortion.2,10 While OCTA devices have useful diagnostic tools, it is important to assess images for the presence of these artifacts before relying on these calculated measurements.
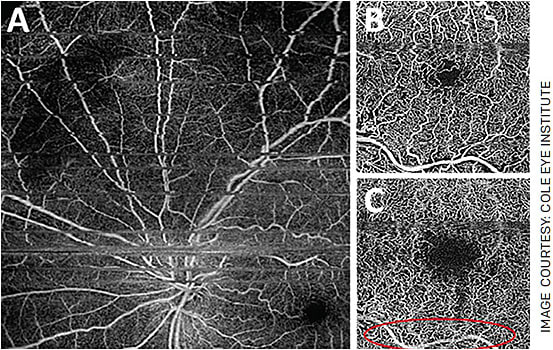
FIGURE 4. Common image artifacts in OCTA include projection artifacts and motion artifacts. Motion and blink artifacts appear as grey or black lines across the image (A) and can result in replication of parts of the image. Notice the superficial retinal vessel (B), which appears in the deep capillary plexus image (C) as a projection artifact.
INNOVATING TO BE EVEN BETTER
OCTA is an exciting and rapidly developing tool that is revolutionizing our ability to image the retina and optic nerve. Ophthalmologists can now obtain incredibly detailed, 3D images of the retinal vasculature within seconds and without the use of invasive dyes.
Despite the benefits of OCTA, its limitations do not allow it to fully replace traditional methods such as FA and ICGA at this time. However, new technology is already being developed to address its pitfalls.
For example, research devices are incorporating technology such as montage protocols, which increase the field of view for OCTA to 100°, and software developments like image averaging and projection-resolved algorithms address some of the common image artifacts encountered.
For the time being, OCTA should serve as an adjunct to FA and allows for more frequent imaging to assist in monitoring disease progression. OM
REFERENCES
- Gabriele ML, Wollstein G, Ishikawa H, et al. Optical coherence tomography: history, current status, and laboratory work. Invest Ophthalmol Vis Sci. 2011;52:2425-2436. Published Apr 14, 2011.
- Sampson DM, Dubis AM, Chen FK, et al. Towards standardizing retinal optical coherence tomography angiography: a review. Light Sci Appl 11, 63 (2022). https://doi.org/10.1038/s41377-022-00740-9
- de Carlo TE, Romano A, Waheed NK, et al. A review of optical coherence tomography angiography (OCTA). Int J Retin Vitr 1, 5 (2015). https://doi.org/10.1186/s40942-015-0005-8
- Ong SS, Patel TP, Singh MS. Optical coherence tomography angiography imaging in inherited retinal diseases. J Clin Med. 2019;8:2078. Published 2019 Nov 28.
- Chalam KV, Sambhav K. Optical coherence tomography angiography in retinal diseases. J Ophthalmic Vis Res. 2016;11:84-92. https://www.ncbi.nlm.nih.gov/pmc/articles/PMC4860994 . Accessed Jun. 8, 2022.
- Sun Z, Yang D, Tang Z, et al. Optical coherence tomography angiography in diabetic retinopathy: an updated review. Eye (Lond). 2021;35:149-161.
- Tsai G, Banaee T, Conti FF, Singh RP. Optical coherence tomography angiography in eyes with retinal vein occlusion. J Ophthalmic Vis Res. 2018;13(3):315-332.
- Yang S, Liu X, Li H, Xu J, Wang F. Optical coherence tomography angiography characteristics of acute retinal arterial occlusion. BMC Ophthalmol. 2019;19:147. Published 2019 Jul 10.
- Tranos P, Karasavvidou E-M, Gkorou O, Pavesio C. Optical coherence tomography angiography in uveitis. Journal of Ophthalmic Inflammation and Infection. 2019;9.
- Spaide RF, Fujimoto JG, Waheed NK. Image artifacts in optical coherence tomography angiography. Retina. 2015;35:2163-2180. https://www.ncbi.nlm.nih.gov/pmc/articles/PMC4712934 . Accessed Jun. 8, 2022.