Corneal endothelial dysfunction remains a significant cause of vision loss throughout the world, whether from trauma, iatrogenic causes, hereditary conditions, such as Fuchs’ endothelial corneal dystrophy, or rejection of a previous corneal transplant. Currently, surgical treatments remain the mainstay of therapy, with endothelial keratoplasty (EK) representing the gold standard of procedures to repair the problem.1 That said, the Eye Bank Association of America Statistical Report shows that endothelial cell failure remains the leading indication for transplantation, representing 56% of all transplants done in the US for 2019. In fact, a total of 30,650 EK procedures were performed last year domestically for the treatment of corneal endothelial disease (Figure 1).2
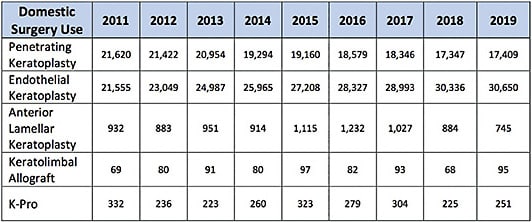
While the US currently has a surplus of cadaveric corneal tissue for EK, this may not last: There is an inverse relationship between age and cornea endothelial density, and the older population that represented one in every eight Americans in 2005 is expected to more than double by 2030.3 The ability to take human cultured endothelial cells (CEC) from cadaveric corneas would significantly offset this potential shortage. After all, CEC allows the use of one donor to treat multiple diseased corneas rather than one donor for one recipient. Additionally, CEC decreases the chances of tissue rejection and graft failures, as the transplantation of cells has much lower immunogenicity than transplanting full-thickness or partial-thickness tissue grafts. But, is CEC a feasible option for the future, possibly overtaking the popularity of EK down the road?
Here, I answer these questions.
Feasible Option
The idea of using CEC as an alternative to EK in the replacement of a diseased corneal endothelium was conceptualized more than 4 decades ago in 1978.4 In 2018, a landmark publication by Kinoshita et al brought it to fruition. Specifically, at 24 weeks after CEC injection supplemented with rho-kinase (ROCK) inhibitor, restoration of corneal transparency with a CEC density of >500 cells per square millimeter was met in 11 of 11 treated eyes (100%; 95% confidence interval [CI], 72 to 100).5 CEC density ranged from 947 to 2833 cells per square millimeter in the 11 eyes (mean density, 1924 cells per square millimeter [95% CI, 1537 to 2312]) and exceeded 1000 cells per square millimeter in 10 eyes and 2000 cells per square millimeter in 6 eyes. (Figure 2).
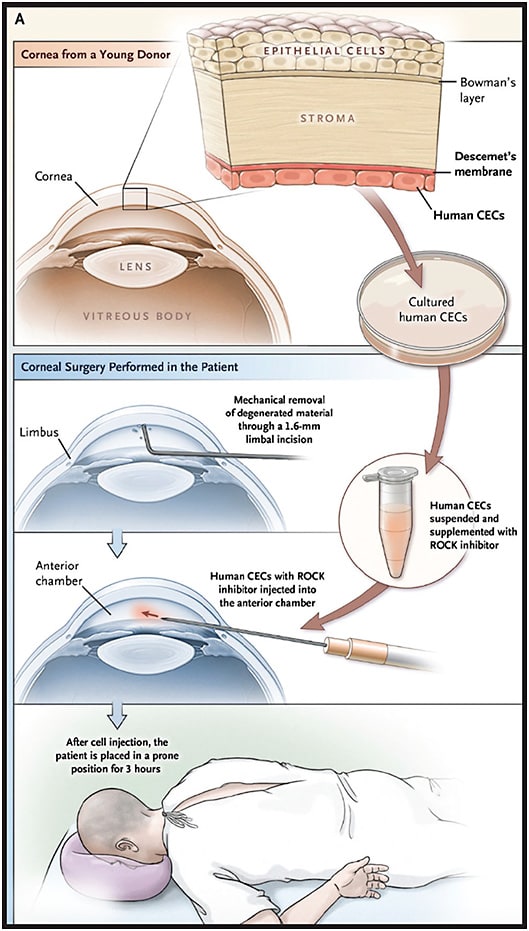
Currently, isolation of CEC involves a two-step technique in which the Descemet membrane (DM) endothelial layer is peeled from the cadaveric donor cornea and then subjected to enzymatic digestion using collagenase, dispase, and trypsin/ethylenediaminetetraacetate. The peeling technique ensures a pure DM with the attached single cell layer of endothelium, as well as supplementation to the media with additives —such as TGF-beta blockage, addition of L-ascorbic acid 2-phosphate, RNA inhibition of p120 activity, inhibition of matrix metalloproteinase activity, and/or fibroblast growth factor withdrawal — that prevent endothelial-mesenchymal transition, which is detrimental to the CEC line.
After isolation of CEC, the line undergoes cultivation and expansion within a culture medium. A large variety of growth-stimulating environments have been developed to support this cellular expansion of CECs. Various media may include endothelial growth medium,2 Dulbecco’s modified Eagle’s medium, human amniotic epithelial cell-conditioned medium, bone marrow-derived mesenchymal stem cell-conditioned medium, and a combination dual media approach between F99 and Endo media. These specialized culture media, together with various growth factors, are shown to support CEC growth and proliferation. The growth factors enhance the CEC’s ability for expansion. Additives, such as human serum, amniotic fluid, and fetal bovine serum, can also stimulate CEC growth. Additional additives to the culture medium include inhibitors to the p160-Rho-associated coiled-coil kinase (ROCK) signaling pathway,6 suspected to lead to cell senescence. Thus, blockage of the pathway with a ROCK inhibitor has been shown to promote the attachment and proliferation of CECs and affords improved survivability of the CEC and continued expansion of the culture line.6
Rho-associated protein kinase inhibitors, such as ripasudil (Glanatec, Kowa), have been shown to demonstrate stimulatory effects on endothelial cell proliferation, adherence, and function. They also inhibit apoptosis and enhance post-wound cellular recovery.7 Human studies show ROCK inhibitor supplementation increases CEC yield within the expanded cell population, especially in younger corneas.
Once CECs have been successfully cultured in vitro, they are delivered to the posterior corneal surface of the recipient, adhere to the DM/stroma, and regulate corneal hydration to promote corneal clearing and transparency. This step can occur through cell injection intracamerally, on an ultra-thin monolayered sheet, or via a cell-carrier system. Direct cell injection requires the patient to lie prone to allow gravity’s assistance in cell adherence. Additionally, ferromagnetic induction or enhancements with substrates, such as a ROCK inhibitor, may be used to promote adherence. Ultra-thin monolayers may be cultured on specially designed surfaces and mobilized upon the adjustment of the surface characteristics to deliver the CECs. The last delivery method involves seeding the CECs onto a transparent biological or synthetic surface prior to transplantation onto the DM-stripped recipient corneal bed. These surfaces have included collagen-based surfaces and silk fibroin polymers.7
It’s important to note that the outcome of a successful primary corneal endothelial culture is also affected by interdonor variability: age of the donor cornea, cause of death, and the length of time between death to preservation to the time for isolation and culture of the CECs. Donors with an acute cause of death, such as a myocardial infarction, have higher levels of intracellular adenosine triphosphate (ATP) for 24 hours after death, allowing for a more optimal metabolic state and better environment for CEC cultivation. Conversely, a donor death due to sepsis results in lower levels of intracellular ATP and a more challenging environment for CEC cultivation with reduced cellular viability.
More Popular Than EK?
For CEC to hit primetime, obstacles must be overcome:
- Expense to cultivate and expand CECs in the laboratory. The costs of additive media, growth factors, and tissue carriers provide more expense than the current gold standard of EK donor grafts. Where will the money come from to fund the research that still needs to be performed for validation of tissue engineering to become mainstream in our eye banks?
- Lack of a reproducible, mainstream protocol. A protocol must be developed for eye banks, so the same product will always be delivered to each corneal surgeon without significant changes in CEC technique. To date, laboratories across the world use different protocols, and nothing yet is standardized with CEC techniques.
Exciting Potential
With there being an inverse relationship between age and corneal density and the older US population expected to grow exponentially over the next decade, CEC would be a welcomed approach for corneal endothelial dysfunction, given its advantages. CP
References
- Lee WB, Jacobs DS, Musch DC, Kaufman SC, Reinhart WJ, Shtein RM. Descemet’s stripping endothelial keratoplasty: safety and outcomes. Ophthalmol. 2009;116(9):1818-1830.
- Eye Bank Association of America. 2019 Statistical Report: Eye Banking Statistical Report: https://restoresight.org/what-we-do/publications/statistical-report/ . Last accessed May 18, 2020.
- Lee WB, Meinecke E, Varnum B. The evolution of eyebanking and corneal transplantation: a symbiotic relationship. Int Ophthalmol Clinics. 2013;53(2):115-129.
- Jumblatt MM, Maurice DM, McCulley JP. Transplantation of tissue-cultured corneal endothelium. Invest Ophthalmol Vis Sci. 1978;17(12):1135-1141.
- Kinoshita S, Koizumi N, Ueno M, et al. Injection of cultured cells with a ROCK inhibitor for bullous keratopathy. N Engl J Med. 2018;378(11):995-1003.
- Okumura N, Ueno M, Koizumi N, et al. Enhancement on primate corneal endothelial cell survival in vitro by a ROCK inhibitor. Invest Ophthalmol Vis Sci. 2009;50(8):3680-3687.
- Soh YQ, Peh GL, Mehta JS. Translational issues for human corneal endothelial tissue engineering. J Tissue Eng Regen Med. 2017; 11: 2425-2442.