Histopathological changes of aqueous outflow
By Haiyan Gong, MD, PhD, FARVO
A primary risk factor for the development and progression of primary open-angle glaucoma (POAG) is elevation of IOP1 resulting from increased resistance to aqueous humor outflow in trabecular outflow pathway.2 An understanding of how to lower IOP in POAG using medical or surgery interventions that target different location(s) along the trabecular outflow pathway begins with an understanding of the anatomy of the aqueous humor drainage pathway in normal eyes and changes in the eyes with POAG.
OVERVIEW OF THE PATHWAY
The vast majority of aqueous humor flows out of the eye through the trabecular outflow pathway. Aqueous exits the anterior chamber through progressively smaller channels of the trabecular meshwork (TM), traverses the monolayer of Schlemm’s canal (SC) endothelial cells to enter a circumferentially oriented SC, then drains from external collector channels (CCs), through the circuitous scleral venous plexus, ultimately joining the episcleral vasculature into the venous system.3 More complicated, aqueous humor that flows out of the eye is segmental, not uniformly circumferential (Figure 1).4-5 Aqueous humor flows preferentially toward the location where the resistance is lower along the outflow pathway. Preferential active outflow was observed often in the nasal and inferior quadrants of the human eye, which are associated with more expanded TM and higher number of CCs.5 The majority of outflow resistance in the TM in normal eyes is generated in the juxtacanalicular connective tissue (JCT)2,6,7 and modulated by the inner wall endothelial cells of SC and their pores.8 However, complete removal of the TM2,9 or precisely removing portions of sclera distal to the outer wall of SC10 suggest that one-third to one-half of the total outflow resistance is located distal to SC.
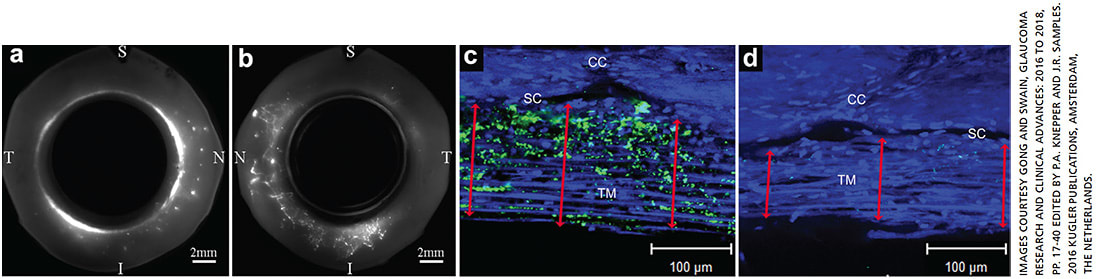
Reduced active flow area was found in acute elevation of IOP11 and in eyes with POAG,12 which was associated with decreased outflow facility. Histopathological changes that cause increased outflow resistance, thus resulting in the reduction of active outflow in the eyes with POAG, can occur in the TM, SC, distal to SC or in all three locations.3
TRABECULAR MESHWORK
In the TM, a progressive age-related loss of TM cells was found in normal eyes, but cellular loss beyond that of normal aging was found in the TM of eyes with POAG.13-14 Fusion of the denuded portions of adjacent trabecular beams was found in eyes with advanced POAG.3 Patients with severe loss of TM cells in advanced glaucoma may not respond well to the new glaucoma drugs that target cytoskeleton of TM cells. Currently, studies are exploring the potential of a cell-based treatment to repopulate the TM with human TM stem cells and restore its function.15-18
An abnormal accumulation of extracellular matrix (ECM) in the TM and JCT was reported in eyes with POAG, whether or not the patients were receiving medical treatment.19-21 An abnormal accumulation of ECM was found to be associated with increasing severity of optic nerve damage,22 but morphometric studies of such specimens have been unable to account for the greater outflow resistance in POAG. Numerous studies have demonstrated that transforming growth factor-β2 (TGF-β2) is elevated in the aqueous humor of patients with POAG.23-26 High levels of TGF-β2 promote ECM formation and inhibit ECM degradation in the TM, both of which contribute to an increase in IOP.27-30
SCHLEMM’S CANAL
In SC, both increased stiffness of the inner wall endothelial cells of SC31-32 and narrowing and collapse of SC33-34 contribute to increased outflow resistance and IOP in POAG. A recent study showed increased stiffness in POAG eyes resided within about 1 μm of the inner-wall surface, while giant vacuole size and density of inner wall endothelial cells of SC were similar between POAG and normal eyes examined in this study, despite lower flow rate through the POAG eyes due to their higher flow resistance. This study concluded that the increased flow resistance of POAG eyes occurs within approximately 1 μm of the surface of the inner wall endothelial cells of SC, resulting from the increased stiffness of the endothelial cells themselves, their immediate underlying extracellular matrix (e.g., basal lamina) or both.32 This increased stiffness of the inner wall of SC and the immediate underlying extracellular matrix may contribute to decreased pore formation in the endothelial cells of SC found in POAG eyes.35-36 Fewer pores decrease the ability of the inner wall cells to permit aqueous humor to enter the lumen of SC. Decreasing the stiffness and increasing the number of pores in the endothelial cells of SC could be a new therapeutic strategy to increase aqueous outflow for glaucoma treatment.
The dimensions of SC are significantly smaller in POAG than in normal eyes histologically.33,37 This finding is confirmed by spectral-domain optical coherence tomographic assessment in patients with POAG.34 Increase in IOP can lead to the progressive narrowing and/or collapse of SC (Figure 2 A-C).11,38 As SC crumples, the outflow resistance grows, and the IOP rises even more.39-40 A shorter scleral spur was found in eyes with POAG, which associated with a higher incidence of SC collapse when compared to age-matched normal eyes.37 Shorter scleral spur in eyes with POAG may be insufficient to hold SC open.37 In one study, the narrowing and collapse of SC could account for nearly half of the decrease in outflow facility in eyes with POAG.33 Additionally, SC became smaller after successful filtration surgery, most likely due to underperfusion of the TM.41 The decrease in the size of SC after successful filtration surgery could make glaucoma more difficult to control, if the filter ultimately fails.
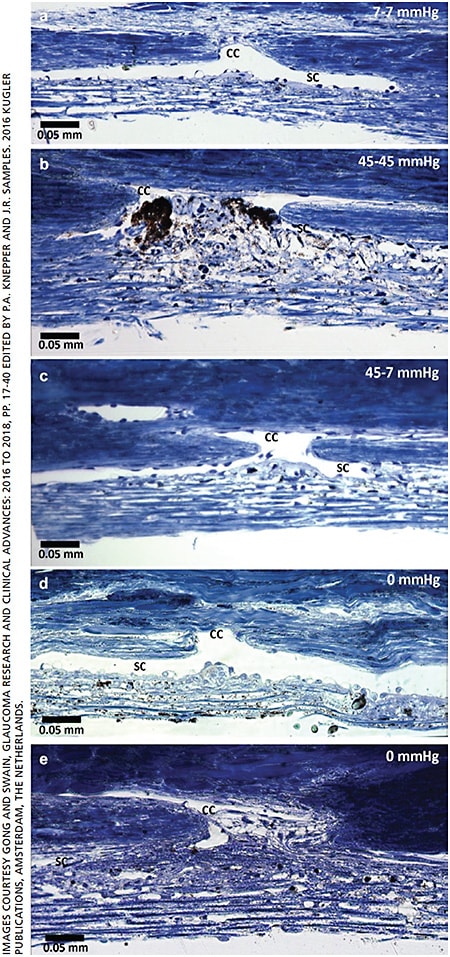
COLLECTOR CHANNELS
In the CCs, herniations block the CC ostia observed in POAG eyes.43-44 When the IOP rises, the TM extends toward the outer wall of SC and leads to the progressive collapse of SC.11,38 Because there is no outer wall of SC in the region of the CC ostia, the JCT and inner-wall tissue herniate into the CC ostia.11 Under experimental conditions in normal eyes, the herniations were reversible when the IOP decreased from high to normal levels.42 Permanent herniations were observed in eyes with POAG, even when the eyes were fixed at zero pressure (Figure 2).43 The obstruction of CCs was also detected in patients with POAG through an evaluation of the fluorescein egress from SC to the episcleral veins and blood reflux from the episcleral veins to SC.4,45 These findings strongly suggest that the obstruction of CCs contributes to increased outflow resistance.
INTRASCLERAL AND EPISCLERAL VEINS
The average width of the intrascleral veins was found to be smaller in glaucoma than normal eyes in an earlier study with a small sample size.46 Obstruction of episcleral veins induces elevation of IOP and glaucoma experimentally.47 Netarsudil (Rhopressa, Aerie Pharmaceuticals) lowers IOP through multiple mechanisms, including dilating episcleral veins and reducing episcleral venous pressure.48-49
CONCLUSION
This article summarizes the histopathological changes that may increase outflow resistance and IOP along the trabecular outflow pathway of eyes with POAG. These histopathological changes play a role in decreasing active outflow in the eyes with POAG. Understanding these changes along each location of the aqueous outflow pathway helps us to improve or restore the impaired aqueous drainage function in the patient with POAG using medical and surgical treatments that target one or multiple affected locations. OM
REFERENCES
- Nemesure B, Honkanen R, Hennis A, et al; Barbados Eye Studies Group. Incident open-angle glaucoma and intraocular pressure. Ophthalmology. 2007;114:1810-1815.
- Grant WM. Experimental aqueous perfusion in enucleated human eyes. Arch Ophthalmol. 1963;69:783-801.
- Gong H, Swain DL. The histopathological changes in the trabecular outflow pathway and their possible effects on aqueous outflow in eyes with primary ppen-angle glaucoma, Glaucoma Research and Clinical Advances: 2016 to 2018, pp. 17-40 Edited by P.A. Knepper and J.R. Samples 2016 Kugler Publications, Amsterdam, The Netherlands.
- Gong H and Francis A: Schlemm’s Canal and Collector Channels as Therapeutic Targets. In Innovations in Glaucoma Surgery, Samples JR and Ahmed I eds. Chapter 1, page 3-25, Springer New York, 2014.
- Cha EDK, Xu J and Gong H: Variations in active outflow along the trabecular outflow pathway. Exp. Eye Res. 2016;146:354-60
- Mäepea O, Bill A. Pressures in the juxtacanalicular tissue and Schlemm’s canal in monkeys. Exp Eye Res. 1992;54:879-883.
- Mäepea O, Bill A. The pressures in the episcleral veins, Schlemm’s canal and the trabecular meshwork in monkeys: effects of changes in intraocular pressure. Exp Eye Res. 1989;49:645-663.
- Johnson M, Shapiro A, Ethier CR, Kamm RD. Modulation of outflow resistance by the pores of the inner wall endothelium. Invest Ophthalmol Vis Sci. 1992;33:1670-1675.
- Rosenquist R, Epstein D, Melamed S, Johnson M, Grant WM. Outflow resistance of enucleated human eyes at two different perfusion pressures and different extents of trabeculotomy. Curr Eye Res. 1989;8:1233-1240.
- Schuman JS, Chang W, Wang N, de Kater AW, Allingham RR. Excimer laser effects on outflow facility and outflow pathway morphology. Invest Ophthalmol Vis Sci. 1999;40:1676-1680.
- Battista SA, Lu Z, Hofmann S, Freddo T, Overby DR, Gong H. Reduction of the available area for aqueous humor outflow and increase in meshwork herniations into collector channels following acute IOP elevation in bovine eyes. Invest Ophthalmol Vis Sci 2008;49:5346-5352.
- Gong L, Cha E and Gong H: Hydrodynamic and Morphological Changes along the Trabecular Outflow Pathway in POAG Eyes, Invest Ophthalmol Vis Sci. 2016;57:5141.
- Alvarado J, Murphy C, Polansky J, Juster R. Age-related changes in trabecular meshwork cellularity. Invest Ophthalmol Vis Sci. 1981;21:714-727.
- Alvarado J, Murphy C, Juster R. Trabecular meshwork cellularity in primary open-angle glaucoma and nonglaucomatous normals. Ophthalmology. 1984;91:564-579.
- Du Y, Roh DS, Mann MM, Funderburgh ML, Funderburgh JL, Schuman JS. Multipotent stem cells from trabecular meshwork become phagocytic TM cells. Invest Ophthalmol Vis Sci 2012;53:1566-1575.
- Abu-Hassan DW, Li X, Ryan EI, Acott TS, Kelley MJ. Induced pluripotent stem cells restore function in a human cell loss model of open-angle glaucoma. Stem Cells 2015;33:751-761.
- Yun H, Lathrop KL, Yang E, et al. A laser-induced mouse model with long-term intraocular pressure elevation. PLoS One 2014;9:e107446.
- Du Y, Yun H, Yang E, Schuman JS. Stem cells from trabecular meshwork home to TM tissue in vivo. Invest Ophthalmol Vis Sci 2013;54:1450-1459.
- Alvarado JA, Yun AJ, Murphy CG. Juxtacanalicular tissue in primary open angle glaucoma and in nonglaucomatous normals. Arch Ophthalmol 1986;104:1517-1528.
- Rohen JW, Lütjen-Drecoll E, Flügel C, Meyer M, Grierson I. Ultrastructure of the trabecular meshwork in untreated cases of primary open-angle glaucoma (POAG). Exp Eye Res. 1993;56:683-692.
- Lütjen-Drecoll E, Shimizu T, Rohrbach M, Rohen JW. Quantitative analysis of ‘plaque material’ in the inner- and outer wall of Schlemm’s canal in normal- and glaucomatous eyes. Exp Eye Res. 1986;42:443-455.
- Gottanka J, Johnson DH, Martus P, Lütjen-Drecoll E. Severity of optic nerve damage in eyes with POAG is correlated with changes in the trabecular meshwork. J Glaucoma. 1997;6:123-132.
- Tripathi RC, Li J, Chan WF, Tripathi BJ. Aqueous humor in glaucomatous eyes contains an increased level of TGF-beta 2. Exp Eye Res. 1994;59:723-727.
- Inatani M, Tanihara H, Katsuta H, et al. Transforming growth factor-beta 2 levels in aqueous humor of glaucomatous eyes. Graefes Arch Clin Exp Ophthalmol. 2001;239:109-113.
- Min SH, Lee TI, Chung YS, Kim HK. Transforming growth factor-beta levels in human aqueous humor of glaucomatous, diabetic and uveitic eyes. Korean J Ophthalmol. 2006;20:162-165.
- Ochiai Y, Ochiai H. Higher concentration of transforming growth factor-beta in aqueous humor of glaucomatous eyes and diabetic eyes. Jpn J Ophthalmo.l 2002;46:249-253.
- Lütjen-Drecoll E. Morphological changes in glaucomatous eyes and the role of TGFbeta2 for the pathogenesis of the disease. Exp Eye Res. 2005;81:1-4.
- Welge-Lüssen U, May CA, Lütjen-Drecoll E. Induction of tissue transglutaminase in the trabecular meshwork by TGF-beta1 and TGF-beta2. Invest Ophthalmol Vis Sci. 2000;41:2229-2238.
- Fuchshofer R, Welge-Lussen U, Lütjen-Drecoll E. The effect of TGF-beta2 on human trabecular meshwork extracellular proteolytic system. Exp Eye Res. 2003;77:757-765.
- Neumann C, Yu A, Welge-Lüssen U, Lütjen-Drecoll E, Birke M. The effect of TGF-beta2 on elastin, type VI collagen, and components of the proteolytic degradation system in human optic nerve astrocytes. Invest Ophthalmol Vis Sci. 2008;49:1464-1472.
- Last JA, Pan T, Ding Y, et al. Elastic modulus determination of normal and glaucomatous human trabecular meshwork. Invest Ophthalmol Vis Sci. 2011;52:2147-2152.
- Vahabikashi A, Gelman A, Dong B, et al. Increased stiffness and flow resistance of the inner wall of Schlemm’s canal in glaucomatous human eyes [published online ahead of print, 2019 Dec 5]. Proc Natl Acad Sci U S A. 2019;116(52):26555-26563.
- Allingham RR, de Kater AW, Ethier CR. Schlemm’s canal and primary open angle glaucoma: correlation between Schlemm’s canal dimensions and outflow facility. Exp Eye Res. 1996;62:101-109.
- Hong J, Xu J, Wei A, et al. Spectral-domain optical coherence tomographic assessment of Schlemm’s canal in Chinese subjects with primary open-angle glaucoma. Ophthalmology. 2013;120:709-715.
- Allingham RR, de Kater AW, Ethier CR, Anderson PJ, Hertzmark E, Epstein DL. The relationship between pore density and outflow facility in human eyes. Invest Ophthalmol Vis Sci 1992;33:1661-1669.
- Johnson M, Chan D, Read AT, et al. The pore density in the inner wall endothelium of Schlemm’s canal of glaucomatous eyes. Invest Ophthalmol Vis Sci. 2002;43:2950-2955.
- Swain DL, Ho J, Lai J, Gong H. Shorter scleral spur in eyes with primary open-angle glaucoma. Invest Ophthalmol Vis Sci. 2015;56:1638-1648.
- Johnstone M, Grant W. Microsurgery of Schlemm’s canal and the human aqueous outflow system. Am J Ophthalmol. 1973;76:906-917.
- Moses RA. Circumferential flow in Schlemm’s canal. Am J Ophthalmol. 1979;88(3 pt 2):585-591.
- Van Buskirk EM. Anatomic correlates of changing aqueous outflow facility in excised human eyes. Invest Ophthalmol Vis Sci. 1982;22:625-632.
- Johnson DH, Matsumoto Y. Schlemm’s canal becomes smaller after successful filtration surgery. Arch Ophthalmol 2000;118:1251-1256.
- Zhu JY, Ye W, Wang T, Gong HY. Reversible changes in aqueous outflow facility, hydrodynamics, and morphology following acute intraocular pressure variation in bovine eyes. Chin Med J (Engl). 2013;126:1451-1457.
- Gong H, Swain DL, Gorantla V, et al. Morphological changes near collector channel ostia in eyes with primary open-angle glaucoma. AGS abstract 2018.
- Hann CR, Vercnocke AJ, Bentley MD, Jorgensen SM, Fautsch MP. Anatomic changes in Schlemm’s canal and collector channels in normal and primary open-angle glaucoma eyes using low and high perfusion pressures. Invest Ophthalmol Vis Sci. 2014;55:5834-5841.
- Grieshaber MC, Pienaar A, Olivier J, Stegmann R. Clinical evaluation of the aqueous outflow system in primary open-angle glaucoma for canaloplasty. Invest Ophthalmol Vis Sci. 2010;51:1498-1504.
- Dovrak-Theobals G, Kirk HQ. Aqueous pathway in some cases of glaucoma. Am J Ophthalmol. 1956;41:11-21.
- Urcola JH, Hernández M, Vecino E. Three experimental glaucoma models in rats: comparison of the effects of intraocular pressure elevation on retinal ganglion cell size and death. Exp Eye Res. 2006 Aug;83:429-37.
- Kazemi A, McLaren JW, Kopczynski CC, et al. The Effects of Netarsudil Ophthalmic Solution on Aqueous Humor Dynamics in a Randomized Study in Humans. J Ocul Pharmacol Ther. 2018;34:380-386.
- Ren R, Li G, Le TD, et al. Netarsudil Increases Outflow Facility in Human Eyes through Multiple Mechanisms. Invest Ophthalmol Vis Sci. 2016;57:6197-6209.
About the Author
Homeostasis at its finest
By Ron Fellman, MD, and Davinder Grover, MD, MPH
Reduction of IOP continues to be the mainstay of glaucoma therapy. However, in a patient destined to develop glaucoma, outflow dysfunction likely is occurring long before the patient manifests elevated IOP. This is best understood from observing glaucoma suspects with a strong family history of glaucoma at the same practice for decades. Their IOP may remain “normal” (and their nerve fiber layer stable) for 20-30 years without any sign of glaucoma then unexpectedly become high at year 31 or so. It is calculated that only one of the 25-30 Schlemm’s canal outlet channels has to function at any one time to manage outflow.1,2 Thus, the reserve of the outflow system is vast, and even though the system loses some of its homeostatic poise, it is likely to maintain a reasonable IOP for quite some time, masking the outflow problem. The pathophysiologic process that caused harmful IOP in the above patient did not occur over night, but instead over decades.
It appears that glaucoma is an even sneakier thief of vision than we previously suspected, because pathologic changes in outflow capacity and nerve fiber viability only become evident clinically in the later stage of disease.
OUR DISADVANTAGE
A considerable disadvantage exists for the ophthalmologist when it comes to discovering early outflow problems before overt pressure elevation, even in glaucoma suspects as noted above. The first obvious reason is detecting elevated pressure may be difficult due to the trickery of diurnal IOP and the lack of readily available 24-hour IOP monitoring. The second reason is the majority of the outflow system is concealed inside the sclera, hidden from ordinary slit lamp view. Reasons three, four and five include serial gonioscopy, optic disc appearance and visual fields; they all may look normal while early outflow pathology smolders. Would measurement of serial outflow resistance be useful in glaucoma suspects? Probably, but it is not a practical test at this juncture and much research still needs to be done. Would the onset of glaucoma be delayed or prevented by lowering IOP at “normal” levels that has serial evidence of change in outflow capacity? We await the answer to that along with the best way to accomplish it.
Another disadvantage, number six, is explained by asking the following question: why is hypotony (pressure low enough to interfere with vision) never seen in a “regular” eye, but elevated IOP is much more common? To maintain sharp vision, the normal outflow system is constructed to avoid hypotony, thus the set point of pressure is on the side of ocular hypertension, not hypotony. Every ophthalmologist who performs glaucoma surgery is familiar with the visual complaints due to low IOP. To avoid hypotony, the system must be (and is) chronically biased towards ocular hypertension and is designed to control it in a fairly narrow range. This at least partly explains why it may be difficult to significantly lower IOP after canal-based MIGS. The homeostatic checks and balances of outflow are extremely effective and robust.
OUR CURRENT UNDERSTANDING
As one awaits precision medicine for glaucoma care (which should include a structure function map of the outflow system), clinical judgment is paramount, especially when it comes to glaucoma surgery. A recent publication by the AGS MIGS task force sheds some light on improved definitions of maximal medical therapy, refractory glaucoma, stage of glaucoma, types of MIGS procedures and many other facts that should allow ophthalmologists and researchers to better study patients who are likely to be the best candidates for MIGS.3
In the meantime, lessons from the laboratory, clinic and operating room continue to improve our understanding of the anatomy and physiology of outflow in the human eye. Based on current knowledge, we believe the following:
- Outflow is mainly segmental, not circumferential.
- Significant distal outflow resistance is present even after eliminating proximal resistance (trabecular meshwork/juxtacanalicular tissue/inner wall of Schlemm’s canal), exemplified by a 360° trabeculotomy (around 40% to 50% resistance still remains).4
- Distal outflow is mainly segmental and favored inferonasally.
- Extracellular matrix turnover is tied to mechano-sensing, elasticity and contractility of the outflow structures.5
- There is a pulsatile nature to outflow with aqueous leaving the eye during systole (aqueous current during diastole is not completely understood).
- Valves that span Schlemm’s canal are involved in the outflow system and work in a pulsatile fashion.6
- Ciliary body tension alters outflow.
- Homeostasis is robust.
- Schlemm’s canal is normally partially collapsed in certain areas.7
- There are normally 25 to 35 collector channel outlets8 that open onto the back wall of Schlemm’s canal.
Many of these findings are based on laboratory observations, but fortunately canal-based MIGS allows a brief intraoperative window of opportunity to gain insight into the living outflow system. Even though this is in glaucomatous eyes, some of the information can surely be extrapolated to normal eyes and can confirm or dispute ex-vivo laboratory research.
CONCLUSION
The outflow system continues to challenge us as it is extremely difficult to evaluate, visualize and alter in vivo. Current studies on outflow and the episcleral venous fluid wave are increasing our ability to better understand outflow patterns in the living eye and are surprisingly consistent with ex vivo laboratory findings.9 We feel strongly that an improved understanding of the outflow system and practical methods for measuring outflow capacity in the clinic will be the holy grail of glaucoma monitoring and detection. Until this is available, we will continue to depend on methods for measuring nerve fiber layer damage, the outcome we strive to avoid. OM
REFERENCES
- Ascher KW. The Aqueous Veins: 1. Physiologic importance of the visible elimination of intraocular fluid. Am J Ophthalmol. 1942;25:1174-209
- McEwen WK. Application of Poiseuille’s law to aqueous outflow. Arch Ophthalmol. 1958, 60:290-294
- Fellman RL, Mattox C, Singh K, Flowers B. American Glaucoma Society Position Paper: Microinvasive Glaucoma Surgery. Ophthalmology Glaucoma. 2020;3:1-5
- Rosenquist R, Epstein D, Melamed S, Johnson M. Grant WM. Outflow resistance of enucleated human eyes at two different perfusion pressures and different extents of trabeculotomy. Curr Eye Res 1989;8:1233-40.
- Carreon T, van der Merwe E, Fellman RL, et al. Aqueous outflow—a continuum from trabecular meshwork to episcleral veins. Progress Retin Eye Res. 2017;57:108-133.
- Xin C, Johnstone M, Wang N, et al. OCT study of mechanical properties associated with trabecuar meshwork and collector channel motion in human eyes. PLoS One. 2016;11(9):e0162048. Published 2016 Sep 6.
- Battista SA, Zhaozeng L, Hofmann S, et al. Reduction of the available area for aqueous humor outflow and increase in meshwork herniations into collector channels following acute IOP elevation in bovine eyes. Invest Ophthalmol Vis Sci.2008;49:5346-5352.
- Dvorak-Theobald G. Schlemm’s canal: its anastomes and anatomic relations. Trans Am Ophthalmol Soc. 1934;32:574-595.
- Fellman RL. Grover DS. Episcleral venous fluid wave in the living human eye adjacent to microinvasive glaucoma surgery (MIGS) supports laboratory research: outflow is limited circumferentially, conserved distally, and favored inferonasally. J Glaucoma 2019;28:139-145.