While clinical examination of the optic disc remains essential to glaucoma diagnosis and management, it is a complex process and difficult to adequately document specific findings in a permanent record.1,2 Even when drawings or notations are documented in a patient’s record, two-dimensional conceptual representations, such as the cup-to-disc ratio, are limited when applied to this complex three-dimensional structure. For example, the edge of the optic “cup” is commonly sloped such that its dimensions vary with the longitudinal position (axial depth) of the measurement plane. It is difficult to identify an axial plane precisely under conditions of live examination (such as binocular indirect ophthalmoscopy) or even within stereoscopic photographs.
Therefore, it is not surprising that even highly trained specialists do not agree with each other, or repeat with high precision, judgments about the degree of glaucomatous structural damage or the likelihood of its progression when viewing stereoscopic color photographs of the optic disc.3-8 So, while most experts are likely to agree on an initial diagnosis, it remains difficult to detect subtle changes in optic disc structure on the basis of clinical examination with or without stereo photos — hence the intense interest over the past two decades in the ongoing development of imaging techniques capable of providing objective, quantitative, reliable measurements of optic disc structure.9
With these proven capabilities, objective imaging techniques have become an integral component of both clinical examination and research in glaucoma.10
CSLT – AN EARLY TECHNIQUE
Among these techniques, confocal scanning laser tomography (CSLT) effectively paved the way. The Heidelberg Retina Tomograph (HRT) ultimately grew to become the most thoroughly implemented and widely adopted platform for performing CSLT and has been incorporated into clinical practice and glaucoma research, including as an ancillary outcome measure for randomized clinical trials.11-16
CSLT provides a high-resolution, two-dimensional map of optic disc surface topography by detecting the strong reflection produced at the interface between the vitreous and the optic disc or retinal tissue. Once an axial reference plane and the optic disc margin are defined, the cup can be defined as that portion inside the disc where its surface lies posterior to the reference plane. Similarly, the neuroretinal rim tissue can be measured with relatively high precision as the area inside the disc that lies anterior to (or “above”) the reference plane.
However, this approach has three potential drawbacks that can affect repeatability and accuracy. First, the disc margin is user defined (subjective) and based on tissue reflectance properties that do not necessarily correspond consistently to specific anatomical structures (i.e., the anatomy defining the disc margin can vary between eyes or even between radial sectors of the same eye).17,18 Second, the rim width measurements are made parallel to the axial reference plane, which does not reflect the geometric orientation of the nerve fibers passing through the rim in the vast majority of eyes.19,20 Third, variability of the axial reference plane ultimately hampers both the precision and accuracy of CSLT measurements and the ability to detect structural changes in glaucoma.21-23
The latter constraint is akin to the problem facing clinicians during live examination and/or evaluation of stereo photos: It is difficult to establish a reliable axial reference plane. Given this inherent limitation common to both approaches, there is only modest agreement between CSLT and expert analysis of stereo photos for detecting progressive structural changes of the optic disc.13,24,25
OCT – TECHNOLOGICAL ADVANTAGES
In this context, one of the fundamental advantages of optical coherence tomography (OCT) is its capability of producing cross-sectional images through tissue with high axial resolution. This allows for the identification of specific anatomical structures deeper within the optic nerve head (ONH) with high precision and accuracy. These capabilities should ultimately solve the previously mentioned reference plane and disc margin problems by enabling those definitions to be based on direct visualization of specific anatomic entities, such as the Bruch’s membrane opening (BMO), the anterior scleral surface or perhaps the anterior scleral canal opening.26-28
Most OCT instruments used in a clinical setting already include fixed scan protocols for glaucoma diagnosis and management, as well as software to report parameters of optic disc neuroretinal rim thickness, rim area, rim volume and, similarly, cup area or volume. OCT measurements of these structural markers already implemented in commercial OCT software packages generally perform well in clinical research studies and enhance diagnostic capability beyond isolated use of stereoscopic disc photos or other OCT parameters.29-33
OCT – LATEST RESEARCH FINDINGS
This remains a very active field of research that continues to develop and produce results that can influence clinical care and commercial instrumentation. Two focal points have shown early promise in clinical research studies.
The first focal point is the ability to visualize and measure aspects of lamina cribrosa anatomy in-depth using OCT.34-38 It has long been thought that the lamina cribrosa is the primary site of injury to retinal ganglion cell axons in glaucoma. OCT provides the ability to measure its depth, thickness, curvature and other aspects of its shape as well as microscopic aspects of lamina cribrosa anatomy such as beam thickness, pore size, pore density and pore shape. This should offer future insights into pathophysiology and the ability to refine estimates of risk for a given eye — that is, to help determine if an eye is relatively more or less susceptible to develop glaucoma or rapidly progressing disease. OCT imaging of the lamina cribrosa for glaucoma assessment is still on the horizon for clinical implementation and thus beyond the scope of this article, but there are excellent recent reviews on this topic.39-41
The second focal point of recent research has been the development of new standards for other OCT-based measurements of optic disc structure as they pertain to glaucoma diagnosis. Perhaps most immediate among them is an approach to measuring neuroretinal rim thickness based on minimum distance mapping. As introduced by Povazay et al42 and subsequently shown by Chen to have meaningful clinical utility,43 ONH rim tissue thickness measurements derived via minimum distance determination have several important advantages. First and foremost is the geometrical relationship to the orientation of axon bundles as they pass through the rim area to enter the scleral canal and exit the globe. The minimum distance approach constrains the thickness measurement to being made at the thinnest point along the rim and as close to being perpendicular to the axon bundles as possible.17,18
This theoretical strength has meaningful clinical benefits, such as avoiding overestimates of rim thickness produced by methods based on less sound geometry. This results in improved diagnostic performance44 and stronger correlations to visual field sensitivity.20,45,46
When ONH rim tissue thickness is measured in an OCT scan as the distance from the BMO point to the nearest point along the optic disc surface, it is commonly termed the BMO-minimum rim width (BMO-MRW, or simply MRW) (Figure 1A, page 27).17,18 The MRW parameter enhances diagnostic performance compared to methods of rim width measurement based on less appropriate geometry, such as implemented in CSLT or older OCT approaches, where the width is determined “horizontally” (within the reference plane) and from an outer edge defined by the clinical disc margin instead of by the BMO.44,46
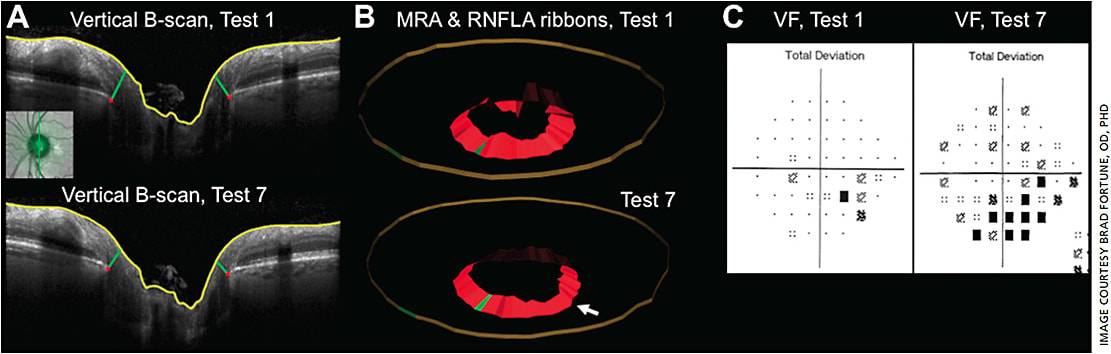
However, optic discs with a larger diameter have a thinner neuroretinal rim for the same number and caliber of axons. Therefore, to have the best possible diagnostic utility, measurements of rim width (i.e. rim thickness) need to be adjusted for disc size.47,48 Alternatively, the total cross-sectional area of rim tissue should be directly proportional to the total number and caliber of axons in each eye and thus unrelated to disc size. Hence the same approach for minimum distance mapping has also been applied to measure the optic disc rim area.45 Gardiner, et al demonstrated that the minimum rim area (MRA) parameter (Figure 1B) exhibited stronger correlation to visual field sensitivity and to peripapillary retinal nerve fiber layer (RNFL) thickness than the HRT- or OCT-derived “horizontal” measurements of rim area.45 Although, the MRW parameter (rim width) correlated equally well to the visual field and RNFL thickness in that study even without adjustment for disc size.45,48 It is likely that disc size has greater impact at the extremes for very small or very large discs or discs of highly myopic eyes.46,49,50 Other similar approaches based on minimum distance mapping have confirmed the advantages it provides for glaucoma diagnostics.51-53
Some of the earliest work in this area was conducted in experimental glaucoma models, which showed that the disc rim tissue thinned earlier and to a greater degree than the peripapillary RNFL tissue.47,54-56 Thus, applying OCT measures of the optic disc rim tissue to glaucoma diagnosis in a cross-sectional, population-based manner may provide additional benefit. However, other work has shown that measurement variability is somewhat larger for MRW and MRA compared with peripapillary RNFL thickness.57 This may be one reason that the correlation with orbital optic nerve axon counts over a wide range of glaucomatous damage is stronger for peripapillary RNFL thickness than for either of these two parameters of the neuroretinal rim.58
Future work in this area will include methods for global optimization of the minimum distance defining rim thickness42,59 rather than the localized method currently employed, although recent work suggests that this will provide only marginal (if any) additional improvement.59 Also, the effect of normal aging on rim parameters such as MRW and MRA will be important to consider and incorporate into commercial instrument reports.60
CONCLUSION
The ability of OCT to provide high-resolution, cross-sectional images through the optic disc tissue enables improved visualization, as well as accurate and precise measurement of anatomical structures important to glaucoma diagnostics and pathophysiology. Important structural targets for OCT include the neuroretinal rim tissue, the BMO, the anterior scleral canal opening and various aspects of lamina cribrosa morphology.
Combined with emerging standards for clinical OCT scan acquisition protocols and morphometric measurements based on the minimum distance approach, these new diagnostic parameters such as MRW and MRA offer meaningful benefits to glaucoma diagnosis and detection of progression. For example, it is likely that in some cases optic disc rim thinning will occur prior to thinning of the RNFL or macular ganglion cell layer, which means OCT measurements like MRW or MRA could enhance early detection of glaucoma. These OCT-based neural rim measurements may also help differentiate glaucoma from other optic neuropathies (which generally exhibit less deformation and “cupping”).
Nevertheless, other structural imaging targets, such as thickness of the peripapillary RNFL and macular inner retinal layers, remain important to detection and management of glaucoma, not least because of their high test–retest repeatability, so they should continue to be used in conjunction with OCT scans of the ONH for maximum efficacy. Ideally, instrument makers will include results of all three target areas in standard glaucoma reports going forward. OM
REFERENCES
- Fremont AM, Lee PP, Mangione CM, et al. Patterns of care for open-angle glaucoma in managed care. Arch Ophthalmol. 2003;121:777-783.
- Quigley HA, Friedman DS, Hahn SR. Evaluation of practice patterns for the care of open-angle glaucoma compared with claims data: the Glaucoma Adherence and Persistency Study. Ophthalmology. 2007;114:1599-1606.
- Varma R, Steinmann WC, Scott IU. Expert agreement in evaluating the optic disc for glaucoma. Ophthalmology. 1992;99:215-221.
- Azuara-Blanco A, Katz LJ, Spaeth GL, Vernon SA, Spencer F, Lanzl IM. Clinical agreement among glaucoma experts in the detection of glaucomatous changes of the optic disk using simultaneous stereoscopic photographs. Am J Ophthalmol. 2003;136:949-950.
- Altangerel U, Bayer A, Henderer JD, Katz LJ, Steinmann WC, Spaeth GL. Knowledge of chronology of optic disc stereophotographs influences the determination of glaucomatous change. Ophthalmology. 2005;112:40-43.
- Parrish RK 2nd, Schiffman JC, Feuer WJ, et al. Test-retest reproducibility of optic disk deterioration detected from stereophotographs by masked graders. Am J Ophthalmol. 2005;140:762-764.
- Jampel HD, Friedman D, Quigley H, et al. Agreement among glaucoma specialists in assessing progressive disc changes from photographs in open-angle glaucoma patients. Am J Ophthalmol. 2009;147:39-44 e31.
- Breusegem C, Fieuws S, Stalmans I, Zeyen T. Agreement and accuracy of non-expert ophthalmologists in assessing glaucomatous changes in serial stereo optic disc photographs. Ophthalmology. 2011;118:742-746.
- Reus NJ, Lemij HG, Garway-Heath DF, et al. Clinical assessment of stereoscopic optic disc photographs for glaucoma: the European Optic Disc Assessment Trial. Ophthalmology. 2010;117:717-723.
- Lin SC, Singh K, Jampel HD, et al. Optic nerve head and retinal nerve fiber layer analysis: a report by the American Academy of Ophthalmology. Ophthalmology. 2007;114:1937-1949.
- Fingeret M, Flanagan JG, Liebmann JM. The Essential HRT Primer. San Ramon, CA: Jocoto Advertising, Inc.; 2005.
- Sharma P, Sample PA, Zangwill LM, Schuman JS. Diagnostic tools for glaucoma detection and management. Surv Ophthalmol 2008;53 Suppl1:S17-32.
- Wollstein G, Garway-Heath DF, Fontana L, Hitchings RA. Identifying early glaucomatous changes. Comparison between expert clinical assessment of optic disc photographs and confocal scanning ophthalmoscopy. Ophthalmology. 2000;107:2272-2277.
- Alencar LM, Bowd C, Weinreb RN, Zangwill LM, Sample PA, Medeiros FA. Comparison of HRT-3 glaucoma probability score and subjective stereophotograph assessment for prediction of progression in glaucoma. Invest Ophthalmol Vis Sci. 2008;49:1898-1906.
- Zangwill LM, Weinreb RN, Beiser JA, et al. Baseline topographic optic disc measurements are associated with the development of primary open-angle glaucoma: the Confocal Scanning Laser Ophthalmoscopy Ancillary Study to the Ocular Hypertension Treatment Study. Arch Ophthalmol. 2005;123:1188-1197.
- Zangwill LM, Jain S, Dirkes K, et al. The rate of structural change: the confocal scanning laser ophthalmoscopy ancillary study to the ocular hypertension treatment study. Am J Ophthalmol. 2013;155:971-982.
- Reis AS, O’Leary N, Yang H, et al. Influence of clinically invisible, but optical coherence tomography detected, optic disc margin anatomy on neuroretinal rim evaluation. Invest Ophthalmol Vis Sci. 2012;53:1852-1860.
- Reis AS, Sharpe GP, Yang H, Nicolela MT, Burgoyne CF, Chauhan BC. Optic disc margin anatomy in patients with glaucoma and normal controls with spectral domain optical coherence tomography. Ophthalmology. 2012.
- Chauhan BC, Burgoyne CF. From clinical examination of the optic disc to clinical assessment of the optic nerve head: a paradigm change. Am J Ophthalmol. 2013;156:218-227 e212.
- Danthurebandara VM, Sharpe GP, Hutchison DM, et al. Enhanced structure-function relationship in glaucoma with an anatomically and geometrically accurate neuroretinal rim measurement. Invest Ophthalmol Vis Sci. 2015;56:98-105.
- Kamal DS, Garway-Heath DF, Hitchings RA, Fitzke FW. Use of sequential Heidelberg retina tomograph images to identify changes at the optic disc in ocular hypertensive patients at risk of developing glaucoma. Br J Ophthalmol. 2000;84:993-998.
- Strouthidis NG, White ET, Owen VM, Ho TA, Garway-Heath DF. Improving the repeatability of Heidelberg retina tomograph and Heidelberg retina tomograph II rim area measurements. Br J Ophthalmol. 2005;89:1433-1437.
- Strouthidis NG, White ET, Owen VM, Ho TA, Hammond CJ, Garway-Heath DF. Factors affecting the test-retest variability of Heidelberg retina tomograph and Heidelberg retina tomograph II measurements. Br J Ophthalmol. 2005;89:1427-1432.
- Vizzeri G, Bowd C, Weinreb RN, et al. Determinants of agreement between the confocal scanning laser tomograph and standardized assessment of glaucomatous progression. Ophthalmology. 2010;117:1953-1959.
- O’Leary N, Crabb DP, Mansberger SL, et al. Glaucomatous progression in series of stereoscopic photographs and Heidelberg retina tomograph images. Arch Ophthalmol. 2010;128:560-568.
- Johnstone J, Fazio M, Rojananuangnit K, et al. Variation of the axial location of Bruch’s membrane opening with age, choroidal thickness, and race. Invest Ophthalmol Vis Sci. 2014;55:2004-2009.
- Vianna JR, Lanoe VR, Quach J, et al. Serial changes in lamina cribrosa depth and neuroretinal parameters in glaucoma: Impact of choroidal thickness. Ophthalmology. 2017;124:1392-1402.
- Luo H, Yang H, Gardiner SK, et al. Factors influencing central lamina cribrosa depth: A multi-center study. Invest Ophthalmol Vis Sci. 2018. In press.
- Mwanza JC, Oakley JD, Budenz DL, Anderson DR, Cirrus Optical Coherence Tomography Normative Database Study G. Ability of cirrus HD-OCT optic nerve head parameters to discriminate normal from glaucomatous eyes. Ophthalmology. 2011;118:241-248 e241.
- Hwang YH, Kim YY. Glaucoma diagnostic ability of quadrant and clock-hour neuroretinal rim assessment using cirrus HD optical coherence tomography. Invest Ophthalmol Vis Sci. 2012;53:2226-2234.
- Loewen NA, Zhang X, Tan O, et al. Combining measurements from three anatomical areas for glaucoma diagnosis using Fourier-domain optical coherence tomography. Br J Ophthalmol. 2015;99:1224-1229.
- Mwanza JC, Warren JL, Budenz DL, Ganglion Cell Analysis Study G. Combining spectral domain optical coherence tomography structural parameters for the diagnosis of glaucoma with early visual field loss. Invest Ophthalmol Vis Sci. 2013;54:8393-8400.
- Larrosa JM, Moreno-Montanes J, Martinez-de-la-Casa JM, et al. A diagnostic calculator for detecting glaucoma on the basis of retinal nerve fiber layer, optic disc, and retinal ganglion cell analysis by optical coherence tomography. Invest Ophthalmol Vis Sci. 2015;56:6788-6795.
- Wollstein G, Paunescu LA, Ko TH, et al. Ultrahigh-resolution optical coherence tomography in glaucoma. Ophthalmology. 2005;112:229-237.
- Srinivasan VJ, Adler DC, Chen Y, et al. Ultrahigh-speed optical coherence tomography for three-dimensional and en face imaging of the retina and optic nerve head. Invest Ophthalmol Vis Sci. 2008;49:5103-5110.
- Kagemann L, Ishikawa H, Wollstein G, et al. Ultrahigh-resolution spectral domain optical coherence tomography imaging of the lamina cribrosa. Ophthalmic Surg Lasers Imaging. 2008;39:S126-131.
- Inoue R, Hangai M, Kotera Y, et al. Three-dimensional high-speed optical coherence tomography imaging of lamina cribrosa in glaucoma. Ophthalmology. 2009;116:214-222.
- Strouthidis NG, Grimm J, Williams GA, Cull GA, Wilson DJ, Burgoyne CF. A Comparison of Optic Nerve Head Morphology Viewed by Spectral Domain Optical Coherence Tomography and By Serial Histology. Invest Ophthalmol Vis Sci. 2009.
- Sigal IA, Wang B, Strouthidis NG, Akagi T, Girard MJ. Recent advances in OCT imaging of the lamina cribrosa. Br J Ophthalmol. 2014;98 Suppl 2:ii34-39.
- Girard MJ, Tun TA, Husain R, et al. Lamina cribrosa visibility using optical coherence tomography: comparison of devices and effects of image enhancement techniques. Invest Ophthalmol Vis Sci. 2015;56:865-874.
- Tan NY, Koh V, Girard MJ, Cheng CY. Imaging of the lamina cribrosa and its role in glaucoma: a review. Clin Exp Ophthalmol. 2018;46:177-188.
- Povazay B, Hofer B, Hermann B, et al. Minimum distance mapping using three-dimensional optical coherence tomography for glaucoma diagnosis. J Biomed Opt. 2007;12:041204.
- Chen TC. Spectral domain optical coherence tomography in glaucoma: qualitative and quantitative analysis of the optic nerve head and retinal nerve fiber layer (an AOS thesis). Trans Am Ophthalmol Soc. 2009;107:254-281.
- Chauhan BC, O’Leary N, Almobarak FA, et al. Enhanced detection of open-angle glaucoma with an anatomically accurate optical coherence tomography-derived neuroretinal rim parameter. Ophthalmology. 2013;120:535-543.
- Gardiner SK, Ren R, Yang H, Fortune B, Burgoyne CF, Demirel S. A method to estimate the amount of neuroretinal rim tissue in glaucoma: comparison with current methods for measuring rim area. Am J Ophthalmol. 2014;157:540-549 e541-542.
- Enders P, Adler W, Kiessling D, et al. Evaluation of two-dimensional Bruch’s membrane opening minimum rim area for glaucoma diagnostics in a large patient cohort. Acta Ophthalmol 2018.
- Patel NB, Sullivan-Mee M, Harwerth RS. The relationship between retinal nerve fiber layer thickness and optic nerve head neuroretinal rim tissue in glaucoma. Invest Ophthalmol Vis Sci 2014;55:6802-6816.
- Amini N, Daneshvar R, Sharifipour F, et al. Structure-Function Relationships in Perimetric Glaucoma: Comparison of Minimum-Rim Width and Retinal Nerve Fiber Layer Parameters. Invest Ophthalmol Vis Sci. 2017;58:4623-4631.
- Enders P, Adler W, Schaub F, et al. Novel Bruch’s Membrane Opening Minimum Rim Area Equalizes Disc Size Dependency and Offers High Diagnostic Power for Glaucoma. Invest Ophthalmol Vis Sci. 2016;57:6596-6603.
- Malik R, Belliveau AC, Sharpe GP, Shuba LM, Chauhan BC, Nicolela MT. Diagnostic Accuracy of Optical Coherence Tomography and Scanning Laser Tomography for Identifying Glaucoma in Myopic Eyes. Ophthalmology. 2016;123:1181-1189.
- Shieh E, Lee R, Que C, et al. Diagnostic Performance of a Novel Three-Dimensional Neuroretinal Rim Parameter for Glaucoma Using High-Density Volume Scans. Am J Ophthalmol. 2016;169:168-178.
- Tsikata E, Lee R, Shieh E, et al. Comprehensive Three-Dimensional Analysis of the Neuroretinal Rim in Glaucoma Using High-Density Spectral-Domain Optical Coherence Tomography Volume Scans. Invest Ophthalmol Vis Sci. 2016;57:5498-5508.
- Fan KC, Tsikata E, Khoueir Z, et al. Enhanced Diagnostic Capability for Glaucoma of 3-Dimensional Versus 2-Dimensional Neuroretinal Rim Parameters Using Spectral Domain Optical Coherence Tomography. J Glaucoma. 2017;26:450-458.
- Strouthidis NG, Fortune B, Yang H, Sigal IA, Burgoyne CF. Longitudinal change detected by spectral domain optical coherence tomography in the optic nerve head and peripapillary retina in experimental glaucoma. Invest Ophthalmol Vis Sci. 2011;52:1206-1219.
- He L, Yang H, Gardiner SK, et al. Longitudinal detection of optic nerve head changes by spectral domain optical coherence tomography in early experimental glaucoma. Invest Ophthalmol Vis Sci. 2014;55:574-586.
- Fortune B, Reynaud J, Hardin C, Wang L, Sigal IA, Burgoyne CF. Experimental Glaucoma Causes Optic Nerve Head Neural Rim Tissue Compression: A Potentially Important Mechanism of Axon Injury. Invest Ophthalmol Vis Sci. 2016;57:4403-4411.
- Gardiner SK, Boey PY, Yang H, Fortune B, Burgoyne CF, Demirel S. Structural Measurements for Monitoring Change in Glaucoma: Comparing Retinal Nerve Fiber Layer Thickness With Minimum Rim Width and Area. Invest Ophthalmol Vis Sci. 2015;56:6886-6891.
- Fortune B, Hardin C, Reynaud J, et al. Comparing Optic Nerve Head Rim Width, Rim Area, and Peripapillary Retinal Nerve Fiber Layer Thickness to Axon Count in Experimental Glaucoma. Invest Ophthalmol Vis Sci. 2016;57:OCT404-412.
- Enders P, Adler W, Schaub F, et al. Optimization Strategies for Bruch’s Membrane Opening Minimum Rim Area Calculation: Sequential versus Simultaneous Minimization. Sci Rep. 2017;7:13874.
- Vianna JR, Danthurebandara VM, Sharpe GP, et al. Importance of Normal Aging in Estimating the Rate of Glaucomatous Neuroretinal Rim and Retinal Nerve Fiber Layer Loss. Ophthalmology. 2015;122:2392-2398.