Optical coherence tomography angiography (OCTA), a no-injection, dye-free, commercially available method of retinal circulation imaging, has seen rapid clinical adoption in ophthalmology. OCTA detects the motion of red blood cells as intrinsic contrast and provides high-resolution images of microvascular networks in addition to structural information.1-3 With the use of OCTA, the optic disc and retinal perfusion could be quantified with high repeatability and reproducibility.2-4 Two OCTA models have received FDA clearance and are commercially available to clinicians in the US: AngioPlex (Carl Zeiss Meditec) and AngioVue (Optovue).
OCTA measures perfusion, which provides information on the metabolic demand and functioning of retinal ganglion cells and nerve fibers. This differs from structural OCT measurements, which relate to the thinning of retinal layers related to the death of retinal ganglion cells and the subsequent loss of cell bodies and axons. Theoretically, OCTA could detect earlier changes in glaucoma, at a stage when some ganglion cells are dysfunctional but not completely lost.
An important advantage of OCTA over traditional dye-based angiography is its three-dimensional nature.2 By segmenting various tissue layers, OCTA could be used for en face visualization and quantification of retinal blood vessels in the superficial vascular complex, which supplies the retinal nerve fiber layer (NFL) and ganglion cell complex.
However, the so-called flow projection artifacts impede investigation of the deeper retinal plexuses. These artifacts are formed by fluctuating shadows cast by flowing blood cells in the more superficial vessels.5,6
Due to the projection artifact, superficial vessels acquire a long tail that spreads into the deeper layers (also called tailing artifact) on cross-sectional OCTA. On en face OCTA, the vascular patterns from more superficial plexuses are duplicated on the deeper layers, making it impossible to obtain a clean image of the deeper vascular plexuses. Furthermore, a false flow signal is projected onto the normally avascular outer retina, which interferes with the accurate identification of choroidal neovascularization.7
Fortunately, the flow projection artifact can be effectively suppressed using an image processing algorithm called “projection-resolved” OCTA (PR-OCTA) that we recently developed at the Casey Eye Institute.5 This algorithm allows visualization of the four human retinal vascular plexuses known from histology, and provides quantitative comparison of vessel densities at each plexus in the retina. Our lab has applied PR-OCTA to detect how glaucoma affects perfusion in the four plexuses.
Acronym glossary
AROC: Area under the receiver operating characteristic
DCP: Deep capillary plexus
DVC: Deep vascular complex
GCC: Ganglion cell complex
GCL: Ganglion cell layer
ICP: Intermediate capillary plexus
INL: Inner nuclear layer
IPL: Inner plexiform layer
NFL: Nerve fiber layer
OCTA: Optical coherence tomography angiography
ONL: Outer nuclear layer
OPL: Outer plexiform layer
PR: Photoreceptor layers
PR-OCTA: Projection-resolved OCTA
RPCP: Radial peripapillary capillary plexus
RPE: Retinal pigment epithelium
RGC: Retinal ganglion cells
SCP: Superficial capillary plexus
SVC: Superficial vascular complex
SVP: Superficial vascular plexus
VD: Vessel density
PROJECTION-RESOLVED OCTA RETINAL VASCULAR ANATOMY
Based on prior primate histology studies, four retinal vascular plexuses can be detected in the macula (Figure 1).8-10 The radial peripapillary capillary plexus (RPCP) has a unique anatomic organization because it runs in parallel with the retinal nerve fiber bundles. The functional significance of the RPCP has been recognized due to its role supplying the NFL.11,12 The superficial vascular plexus (SVP), which is supplied by the central retinal artery, is composed of larger arteries, arterioles, capillaries, venules and veins, vessels primarily in the ganglion cell layer (GCL). There are two deeper capillary networks above and below the inner nuclear layer (INL) referred to as the intermediate capillary plexus (ICP) and deep capillary plexus (DCP), respectively, which are supplied by vertical anastomoses from the superficial vascular plexus.8,10
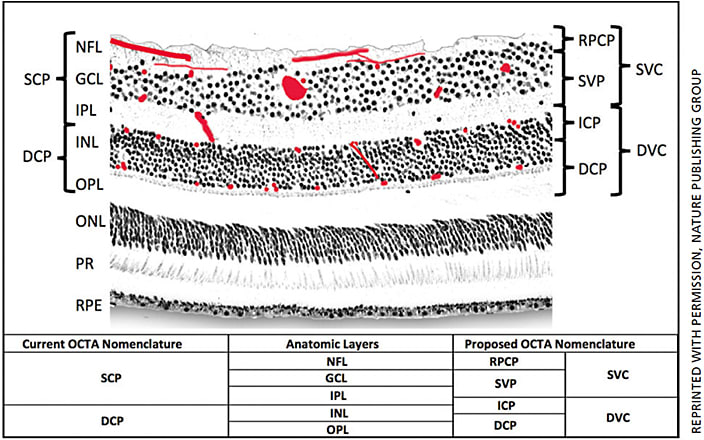
PR-OCTA allows us to study the normal retinal vasculature in vivo with better depth resolution than previously possible. This montage en face PR-OCTA (Figure 2A) from one healthy subject demonstrates the typical angiographic appearance of the inner retina with both large and small vessels. Overlaying the flow signal onto a structural OCT image (Figure 2B), the specific depth and relative breadths of the four vascular plexuses are easily visualized. The radial peripapillary capillary plexus has long radial capillaries that run parallel with the nerve fiber bundles, whereas the SVP consists of a mixture of large and small vessels (Figure 3). In the macula, the SVP has a centripetally branching pattern that terminates at the capillary ring around the fovea avascular zone (Figure 3). The largest arcade vessels interweave between the NFL and ganglion cell layer, and thus are captured in both the RPCP and SVP slabs. The intermediate capillary plexus and deep capillary plexus consist of thin layers of capillaries arranged in lobular patterns without directional preference.7
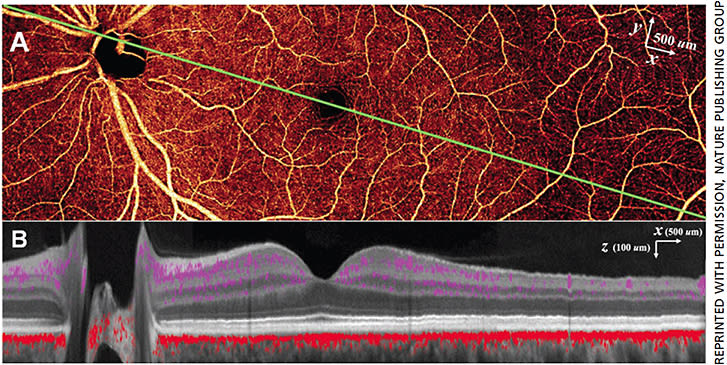
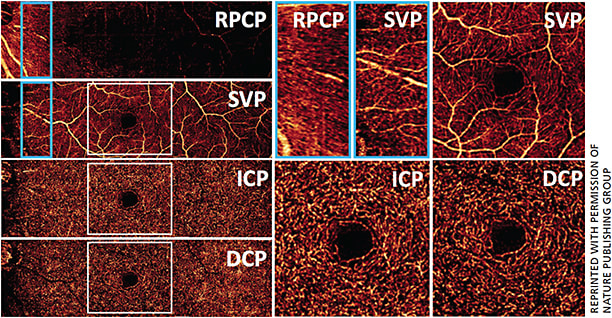
OCTA IN GLAUCOMA
OCTA of the optic disc and peripapillary retinal perfusion has been studied for glaucoma diagnosis and evaluation.3,4,13 In an initial study, the optic disc perfusion was quantified as flow index and found 25% reduction in the glaucoma group.4 In a subsequent pilot study, the reduced peripapillary retinal perfusion in glaucomatous eyes was visualized as focal defects. The peripapillary retinal flow index and vessel density (VD) in glaucomatous eyes were significantly lower than those in normal eyes. In a study with a larger sample size, decreased VD in retinal NFL was found significantly associated with the severity of visual field damage. OCTA parameters showed less floor effect and higher correlation with visual field than retinal NFL thickness.13 These results demonstrate that OCTA technology has the potential for improving glaucoma monitoring, even in eyes with more advanced disease in which the ability to detect progression based on further structural thinning is limited because of the “floor effect” of the structural parameters.
However, it’s still uncertain how glaucoma affects different plexuses in the retina. On conventional OCTA, the vascular patterns from more superficial plexuses are duplicated on the deeper layers, making it impossible to obtain a clean image of the deeper vascular plexuses. As the above figures indicate, PR-OCTA could detect the different perfusion changes in the plexuses in glaucoma. In the peripapillary retina, the most superficial plexus is RPCP, which supplies the NFL (Figure 3). The RPCP VD showed a significant reduction in glaucoma-suspect eyes and glaucomatous eyes.14 Since glaucoma damages retinal ganglion cells (RGC) and approximately 1/3 of RGCs reside in the macula, imaging macular perfusion should be important for glaucoma evaluation. We applied PR-OCTA to investigate plexus-specific macular perfusion changes in glaucoma patients.15 In both normal eyes and glaucomatous eyes, the PR-OCTA and conventional OCTA SVC angiograms differ in the intermediate capillary plexus and deep capillary plexus. In conventional OCTA images, vessel patterns in the SVC are duplicated in the ICP and DCP. In PR-OCTA, the ICP and DCP are pure capillary layers of uniform vessel caliber and density. An inferior arcuate area of capillary dropout could be visualized in the SVC on both PR-OCTA and conventional OCTA. The inferior arcuate defect is artifactually projected onto the ICP in conventional OCTA, but the cleaner PR-OCTA showed that the ICP and DCP are both spared. Statistical analyses showed that SVC VD in the glaucoma group was significantly lower than the age-matched normal control group. There was no significant difference in ICP and DCP VD between the two groups. We also found that by focusing on the SVC VD we improved glaucoma diagnosis and evaluation. Compared to the all-plexus VD, SVC VD had better diagnostic accuracy and better correlation with visual field (VF) sensitivity. Correlation between macular SVC VD and VF sensitivity was greater than the correlation between GCC thickness and VF sensitivity. This finding concurs with previous investigations in the peripapillary region, which showed OCTA-VF correlation to be better than structural OCT-VF correlation. OCTA has the potential to be a better predictor of VF defects/progression than structural OCT.
SUMMARY
PR-OCTA removes projection artifacts in the deeper retinal plexuses and allows accurate localization of vascular changes. In glaucoma, reduction of capillary density is found in the RPCP and in the macular SVC. OCTA measurements in these plexuses are highly correlated with VF loss and could potentially improve glaucoma diagnosis and monitoring. OM
REFERENCES
- Jia Y, Tan O, Tokayer J, et al. Split-spectrum amplitude-decorrelation angiography with optical coherence tomography. Optics Express. 2012;20:4710-4725.
- Jia Y, Bailey ST, Hwang TS, et al. Quantitative optical coherence tomography angiography of vascular abnormalities in the living human eye. Proc Natl Acad Sci U S A. May 05 2015;112:E2395-2402.
- Liu L, Jia Y, Takusagawa HL, et al. Optical Coherence Tomography Angiography of the Peripapillary Retina in Glaucoma. JAMA Ophthalmol. 2015;133:1045-1052.
- Jia Y, Wei E, Wang X, et al. Optical coherence tomography angiography of optic disc perfusion in glaucoma. Ophthalmology. 2014;121:1322-1332.
- Zhang M, Hwang TS, Campbell JP, et al. Projection-resolved optical coherence tomographic angiography. Biomed Opt Express. 2016;7:816-828.
- Liu L, Gao SS, Bailey ST, et al. Automated choroidal neovascularization detection algorithm for optical coherence tomography angiography. Biomed Opt Express. 2015;6:3564-3576.
- Campbell JP, Zhang M, Hwang TS, et al. Detailed vascular anatomy of the human retina by projection-resolved optical coherence tomography angiography. Sci Rep. 2017;7:42201.
- Provis JM. Development of the primate retinal vasculature. Prog Retin Eye Res. 2001;20:799-821.
- Snodderly DM, Weinhaus RS, Choi JC. Neural-vascular relationships in central retina of macaque monkeys (Macaca fascicularis). J Neurosci. 1992;12:1169-1193.
- Stone J, van Driel D, Valter K, Rees S, Provis J. The locations of mitochondria in mammalian photoreceptors: relation to retinal vasculature. Brain Res. 2008;1189:58-69.
- Henkind P. Radial peripapillary capillaries of the retina. I. Anatomy: human and comparative. Br J Ophthalmol. 1967;51:115-123.
- Alterman M, Henkind P. Radial peripapillary capillaries of the retina. II. Possible role in Bjerrum scotoma. Br J Ophthalmol. 1968;52:26-31.
- Yarmohammadi A, Zangwill LM, Diniz-Filho A, et al. Relationship between optical coherence tomography angiography vessel density and severity of visual field loss in glaucoma. Ophthalmology. 2016;123:2498-2508.
- Yarmohammadi A, Zangwill LM, Diniz-Filho A, et al. Optical coherence tomography angiography vessel density in healthy, glaucoma suspect, and glaucoma eyes. Invest Ophthalmol Vis Sci. 2016;57:OCT451-459.
- Takusagawa HL, Liu L, Ma KN, et al. Projection-resolved optical coherence tomography angiography of macular retinal circulation in glaucoma. Ophthalmology. 2017 in press.