Noncontact methods of crossectional bioimaging with optical coherence tomography (OCT) have become an integral part of the evaluation, management and monitoring of a wide range of retinal pathologies.1 For example, spectral-domain OCT (SD-OCT) has provided improvement in resolution and speed of acquisition, which allows for more detailed visualization of vitreoretinal pathology.2
The role of OCT in our space seems unceasing in its expansion. In addition to its clinical management role, OCT imaging now has two more – in pre-operative surgical planning and postoperative evaluation, especially with regard to epiretinal membranes, macular holes, rhegmatogenous and tractional retinal detachments.3-8
OCT continues evolving and improving, becoming ever more helpful intraoperatively. Here’s a look at where the technology stands now.
THE ROAD TO INTRA-OPERATIVE OCT (iOCT)
The conventional table-top OCT unit has always required upright patient positioning and cooperation, so it cannot be used on supine patients in the operative suite.
That changed in 2007 when a portable, handheld SD-OCT scanner (Bioptigen, Inc.) came on the market, allowing imaging of supine patients. It is primarily used in operative suites during exams of anesthetized pediatric patients who have various conditions such as retinopathy of prematurity, albinism and shaken baby syndrome.9-12 Another commonly used handheld system is the iVue (Optovue).13-14
Intraoperative handheld OCT has allowed immediate evaluation of retinal contour, membranes and macular holes prior to and after surgical intervention. Multiple studies have reported feasibility of handheld iOCT to alter surgical decision-making, for example in cases requiring additional membrane peeling to achieve macular hole closure.15,16 However, this device configuration requires pausing of surgical procedures to acquire images, and that portends a higher risk of contaminating the surgical field.
The need to decrease image capture time, ensure sterility and improve reproducibility led to development of microscope-mounted iOCT devices.17-19 These devices allowed for easier alignment of the system, but real-time visualization of the tissue and tissue-instrument interactions were not possible until development of microscope-integrated iOCT (MiOCT) devices.19-21 The optical path of the MiOCT system incorporates into the common optical pathway of the surgical microscope, which allows improved targeting and tracking of the scan beam and achieves parfocal and coaxial OCT imaging with the surgical view.
MiOCT OPTIONS AND BENEFITS
In the first publication of MiOCT use in vitreoretinal surgery, Cynthia Toth, MD, at Duke University, and co-investigators described a custom prototype system: a research OCT integrated with a commercially available operating microscope.22 Other early prototypes include Cirrus SD-OCT (Carl Zeiss Meditec) using the Zeiss OPMI VISU 200 surgical microscope,18 and EnFocus (Leica Microsystems/Bioptigen) iOCT.23 Since 2010 we have seen significant advances in software and hardware of MiOCT systems. The FDA has approved three systems: EnFocus, used with a Leica surgical microscope,23 Haag-Streit iOCT, which is integrated through a microscope side-port,24 and RESCAN 700 (Zeiss), which is built on the Lumera 700 microscope platform.25-27
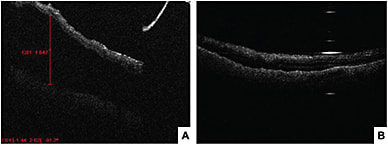
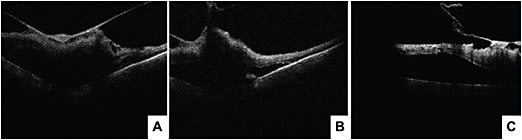
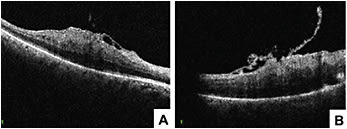
Recent studies examined MiOCT’s use for different conditions, such as epiretinal membrane, vitreomacular traction, macular hole and retinal detachment.28-31 During epiretinal membrane peels, MiOCT allows visualization of the distance growing between the RPE and the ellipsoid zone and the focal areas of retinal elevation at the peel initiation sites.31 However, it remains to be determined whether these intraoperative changes lead to significant functional changes. In macular hole surgery, anatomical success was associated with configuration of macular hole and changes in macular hole geometry seen with MiOCT.30 In vitreomacular traction repair procedures, MiOCT has allowed for assessment of the strength of vitreomacular adhesions and visualization of unroofed cysts, subclinical full-thickness macular hole development and incomplete peeling of membranes. Intraoperative identification of these subclinical changes may change the surgical approach, such as opting for a gas tamponade and potentially preventing the need for future reoperations.29 In retinal detachment surgery, MiOCT allows detection of residual subretinal fluid and can assist in completion of fluid air exchange. Real-time visualization of the planes may also help achieve more precise delamination and segmentation in tractional retinal detachment surgeries.
IT’S FEASIBLE
Many early studies of iOCT were small retrospective case reports. The PIONEER study was the first large prospective study to evaluate feasibility and utility of iOCT, specifically microscope-mounted SD-OCT (Bioptigen).32 Surgeons in this study reported that their approach in retinal membrane peeling procedures was changed by iOCT in 8% of cases.32
It showed that iOCT was feasible in 92% of cases. Per surgeon reports, it was most useful for membrane peeling procedures, providing valuable information, such as presence of residual membrane, in 65% of cases and leading to a change in surgeon’s decision-making in 35% of cases.23 The RESCAN 700 portion of the DISCOVER study similarly demonstrated the feasibility of real-time iOCT in ophthalmic surgery.27
In the RESCAN 700 system, the real-time OCT images are projected on a heads-up display, which allows the surgeon to manipulate scan length, location and angle through video monitor display or the foot pedal control. This study showed that imaging was successfully obtained in 99% of cases and provided new and different information to the surgeon in 19% of cases.27
STILL SOME KINKS TO WORK OUT
Current MiOCT systems have some limitations that require further software and hardware changes. Because there is a lack of OCT-compatible surgical instrumentation, most instruments lead to light scattering and shadowing, limiting to some degree the real-time visualization of instrument-retina areas of contact. The amount of shadowing varies, depending on instrument thickness, material and relative orientation to the optical axis of the OCT. Difficulties with visualizing the scan directly below the area of contact often leave tissue anatomy changes as a more valuable immediate feedback source.28 Development of instruments that minimize scatter and shadowing will allow for more precise tissue manipulation. Software algorithms may assist in software-based processing of the image to minimize shadowing as well as localize the beam to the area of interest. Spatial compounding of B-scans can enhance visualization of the tissue past the instrument.19
In systems with a heads-up display, surgical oculars limit the size of OCT images and the visual field. On the other hand, an external monitor with OCT images requires the surgeon to look away from the surgical field. Additionally, MiOCT systems achieve optimal scans of the macula and posterior pole, with deterioration of image while scanning peripheral retina. There are reports of intraocular probe-type OCTs that may be used to scan target tissue anywhere in the eye.33-36 However, these reports are limited to animal studies or pilot clinical cases.
A very recent development in MiOCT technology is real-time volumetric imaging that can achieve four-dimensional visual feedback of volumes through time (4D MiOCT).37 Instead of real-time iOCT imaging being limited by live B-scans, which prevents visualization of continuous and complete instrument motion, 4D MiOCT acquires, processes and renders volumes in real time. This allows for enhanced visualization of tissue deformation and decreases the need for constant tracking of the moving object. 4D MiOCT, developed at Duke University is not commercially available.
ADDING VALUE
Multiple studies demonstrate the feasibility and value of MiOCT in vitreoretinal surgery. As MiOCT systems offer the surgeon immediate image-guidance, they may improve the surgeon’s judgment, knowledge and decision-making. This technology will provide better understanding of effects of surgical manipulation on the tissues and possibly allow us to explain and predict variations in postoperative visual outcomes. Despite the changes in surgical decision-making reported in MiOCT studies, randomized control trials are needed to evaluate the effect of those changes on long-term patient outcomes. Furthermore, additional studies are needed to evaluate which surgical procedures would benefit the most from iOCT use. In addition to routine vitreoretinal surgical procedures, MiOCT may be beneficial in the future in regenerative and gene therapy, allowing for precise delivery of a therapeutic agent.
Improvement in OCT aiming and tracking, optimization of heads-up display or an external monitor to maximize feedback while minimizing distractions, and development of OCT-compatible instruments will all promote further integration of MiOCT and add value during surgical procedures. OM
REFERENCES
- Huang D, Swanson EA, Lin CP, et al. Optical coherence tomography. Science. 1991;254:1178-1181.
- Gabriele ML, Wollstein G, Ishikawa H, et al. Three dimensional optical coherence tomography imaging: advantages and advances. Prog Retin Eye Res. 2010;29:556-579.
- Jumper JM, Gallemore RP, McCuen BW 2nd, Toth CA. Features of macular hole closure in the early postoperative period using optical coherence tomography. Retina. 2000;20:232-237.
- Ripandelli G, Coppe A, Bonini S, et al. Morphological evaluation of full-thickness idiopathic macular holes by optical coherence tomography. Eur J Ophthalmol. 1999;9:212-216.
- Mikajiri K, Okada AA, Ohji M, et al. Analysis of vitrectomy for idiopathic macular hole by optical coherence tomography. Am J Ophthalmol. 1999;128:655-657.
- Falkner-Radler CI, Glittenberg C, Hagen S, et al. Spectral-domain optical coherence tomography for monitoring epiretinal membrane surgery. Ophthalmology. 2010;117:798-805.
- Massin P, Allouch C, Haouchine B, et al. Optical coherence tomography of idiopathic macular epiretinal membranes before and after surgery. Am J Ophthalmol. 2000;130:732-739.
- Gallemore RP, Jumper JM, McCuen BW 2nd, et al. Diagnosis of vitreoretinal adhesions in macular disease with optical coherence tomography. Retina. 2000;20:115-120.
- Scott AW, Farsiu S, Enyedi LB, et al. Imaging the infant retina with a hand-held spectral-domain optical coherence tomography device. Am J Ophthalmol. 2009;147:364-373.e2.
- Muni RH, Kohly RP, Sohn EH, Lee TC. Hand-held spectral domain optical coherence tomography finding in shaken-baby syndrome. Retina. 2010;30(4 suppl):S45-S50.
- Chong GT, Farsiu S, Freedman SF, et al. Abnormal foveal morphology in ocular albinism imaged with spectral-domain optical coherence tomography. Arch Ophthalmol. 2009;127:37-44.
- Muni RH, Kohly RP, Charonis AC, Lee TC. Retinoschisis detected with handheld spectral-domain optical coherence tomography in neonates with advanced retinopathy of prematurity. Arch Ophthalmol. 2010;128:57-62.
- Riazi-Esfahani M, Khademi MR, Mazloumi M, et al. Macular Surgery Using Intraoperative Spectral Domain Optical Coherence Tomography. J Ophthalmic Vis Res. 2015;10:309-315.
- Branchini LA, Gurley K, Duker JS, Reichel E. Use of Handheld Intraoperative Spectral-Domain Optical Coherence Tomography in a Variety of Vitreoretinal Diseases. Ophthalmic Surg Lasers Imaging Retina. 2016;47:49-54.
- Dayani PN, Maldonado R, Farsiu S, Toth CA. Intraoperative use of handheld spectral domain optical coherence tomography imaging in macular surgery. Retina. 2009;29:1457-1468.
- Wykoff CC, Berrocal AM, Schefler AC, et al. Intraoperative OCT of a full-thickness macular hole before and after internal limiting membrane peeling. Ophthalmic Surg Lasers Imaging. 2010;41:7-11.
- Ray R, Barañano DE, Fortun JA, et al. Intraoperative microscope-mounted spectral domain optical coherence tomography for evaluation of retinal anatomy during macular surgery. Ophthalmology. 2011;118:2212-2217.
- Binder S, Falkner-Radler CI, Hauger C, et al. Feasibility of intrasurgical spectral-domain optical coherence tomography. Retina. 2011;31:1332-1336.
- Ehlers JP, Tao YK, Farsiu S, et al. Visualization of real-time intraoperative maneuvers with a microscope-mounted spectral domain optical coherence tomography system. Retina. 2013;33:232-236.
- Hahn P, Carrasco-Zevallos O, Cunefare D, et al. Intrasurgical Human Retinal Imaging With Manual Instrument Tracking Using a Microscope-Integrated Spectral-Domain Optical Coherence Tomography Device. Transl Vis Sci Technol. 2015;4:1.
- Hahn P, Migacz J, O’Connell R, et al. Unprocessed real-time imaging of vitreoretinal surgical maneuvers using a microscope-integrated spectral-domain optical coherence tomography system. Graefes Arch Clin Exp Ophthalmol. 2013;251:213-220.
- Tao YK, Ehlers JP, Toth CA, Izatt JA. Intraoperative spectral domain optical coherence tomography for vitreoretinal surgery. Opt Lett. 2010;35:3315-3317.
- Runkle A, Srivastava SK, Ehlers JP. Microscope-Integrated OCT Feasibility and Utility With the EnFocus System in the DISCOVER Study. Ophthalmic Surg Lasers Imaging Retina. 2017;48:216-222.
- Steven P, Le Blanc C, Velten K, et al. Optimizing descemet membrane endothelial keratoplasty using intraoperative optical coherence tomography. JAMA Ophthalmol. 2013;131:1135-1142.
- Pfau M, Michels S, Binder S, Becker MD. Clinical Experience With the First Commercially Available Intraoperative Optical Coherence Tomography System. Ophthalmic Surg Lasers Imaging Retina. 2015;46:1001-1008.
- Ehlers JP, Kaiser PK, Srivastava SK. Intraoperative optical coherence tomography using the RESCAN 700: preliminary results from the DISCOVER study. Br J Ophthalmol. 2014;98:1329-1332.
- Ehlers JP, Goshe J, Dupps WJ, et al. Determination of feasibility and utility of microscope-integrated optical coherence tomography during ophthalmic surgery: the DISCOVER Study RESCAN Results. JAMA Ophthalmol. 2015;133:1124-1132.
- Ehlers JP, Ohr MP, Kaiser PK, Srivastava SK. Novel microarchitectural dynamics in rhegmatogenous retinal detachments identified with intraoperative optical coherence tomography. Retina. 2013;33:1428-1434.
- Ehlers JP, Tam T, Kaiser PK, et al. Utility of intraoperative optical coherence tomography during vitrectomy surgery for vitreomacular traction syndrome. Retina. 2014;34:1341-1346.
- Ehlers JP, Xu D, Kaiser PK, et al. Intrasurgical dynamics of macular hole surgery: an assessment of surgery-induced ultrastructural alterations with intraoperative optical coherence tomography. Retina. 2014;34:213-221.
- Ehlers JP, Roth B, Kaiser PK, et al. Assessment of microarchitectural retinal dynamics of epiretinal membrane surgery utilizing intraoperative optical coherence tomography. Macula Society Annual Meeting; Dana Point, CA. 2013.
- Ehlers JP, Dupps WJ, Kaiser PK, et al. The Prospective Intraoperative and Perioperative Ophthalmic ImagiNg With Optical CoherEncE TomogRaphy (PIONEER) Study: 2-Year Results. Am J of Ophthalmol. 2014;158:999–1007.
- Han S, Sarunic MV, Wu J, et al. Handheld forward-imaging needle endoscope for ophthalmic optical coherence tomography inspection. J Biomed Opt. 2008;13:020505.
- Ren J, Gille HK, Wu J, Yang C. Ex vivo optical coherence tomography imaging of collector channels with a scanning endoscopic probe. Invest Ophthalmol Vis Sci. 2011;52:3921-3925.
- Zhao M, Huang Y, Kang JU. Sapphire ball lens-based fiber probe for common-path optical coherence tomography and its applications in corneal and retinal imaging. Opt Lett. 2012;37:4835-4837.
- Asami T, Terasaki H, Ito Y, et al. Development of a Fiber-Optic Optical Coherence Tomography Probe for Intraocular Use. Invest Ophthalmol Vis Sci. 2016;57:OCT568-74.
- Carrasco-Zevallos OM, Keller B, Viehland C, et al. Optical Coherence Tomography for Retinal Surgery: Perioperative Analysis to Real-Time Four-Dimensional Image-Guided Surgery. Invest Ophthalmol Vis Sci. 2016;57:OCT37-50.