OCTA: motion tells the tale
With angiography, increased speed allows an insightful look at the retina’s behavior.
By David Huang, MD, PhD; Yali Jia, PhD; Simon S. Gao, PhD; Steven T. Bailey, MD; Thomas S. Hwang, MD
Optical coherence tomography (OCT), as an imaging modality, is well matched to ophthalmology because of its unique ability to resolve thin anatomic layers in the retina.1 It has been the most common ophthalmic imaging procedure used for more than a decade. Commercial OCT technology has improved tremendously since its initial introduction, with speed increasing from 0.1 to 100 kHz over little more than two decades. This increased speed makes it possible to detect blood flow by comparing consecutive cross-sectional images for changes in either the amplitude or phase of reflected light. This qualitative new development in OCT is called OCT angiography (OCTA).
Advantages Of OCTA
Because OCTA uses the motion of blood cells as intrinsic contrast, no dye injection is required, making it completely noninvasive. Compared to conventional fluorescein angiography (FA) and indocyanine green angiography (ICGA), OCTA has several advantages. OCT is three dimensional, so the choroidal circulation can be viewed separately from the retinal circulation, and the several plexuses within the retina can be viewed separately as well. Segmentation of the 3D OCTA also enables identification of neovascular networks by their locations in normally avascular layers. Furthermore, OCTA, introduced to the marketplace two years ago, provides higher capillary contrast than fluorescein angiography and ICGA because it is not affected by leakage and less affected by hemorrhage or other media opacity. Therefore, clinicians can more easily identify capillary dropout on OCTA and obtain automated computer measurement of vessel density and any nonperfusion area.
Our laboratory, the Center for Ophthalmic Optics and Lasers* developed an efficient OCTA algorithm called the split-spectrum amplitude-decorrelation angiography (SSADA) that enables a high-quality OCTA scan to be obtained on a commercial 70 kHz spectral domain OCT system in 3 seconds.2,3 The SSADA technology led to the AngioVue (Optovue, Inc.), the first clinical OCTA system; it received FDA approval earlier this year. Our experience with the AngioVue system is evident in the examples given in this article. However, the approaches described are applicable to other OCTA systems on the market.
OCT and neovascular AMD
OCT has become indispensable in the management of neovascular age-related macular degeneration (AMD) because it can measure retinal fluid accumulation, which is closely linked to the activity of choroidal neovascularization (CNV) and vision quality. Using the same OCT hardware, clinicians can now directly visualize CNV with OCTA. On en face OCTA (Figure 1), one can see CNV as a vascular network in the normally avascular outer retinal slab (defined to include retinal pigment epithelium [RPE] and Bruch’s membrane). However, the outer retinal OCTA contains a shadow graphic projection of inner retinal vascular patterns (Figure 1 top), making the detection of CNV more difficult.
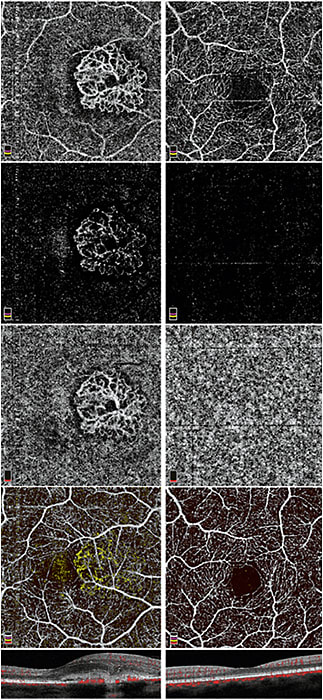
Figure 1. Comparison of an eye with type 2 CNV (left column) with a normal eye (right column). En face images cover a 3×3 mm area. Top row: en face OCTA of the outer retinal slab (including RPE and Bruch’s membrane). Second row: en face OCTA of the outer retina with subtraction of projection artifact from inner retina. Third row: en face OCTA of the choriocapillaris slab. Fourth row: color composite en face OCTA. Inner retinal circulation is in white; outer retinal (CNV) circulation is in yellow. Bottom row: Color cross-sectional OCTA across the center of the CNV, where the flow signal (red) is overlaid on structural OCT. The CNV extends above the RPE, which makes it type 2.
Several solutions exist to detecting these inner vascular patterns.
Algorithms, halos and color composites
One option is to apply the projection subtraction algorithm that is largely effective in removing the inner retinal vascular pattern;4,5 however, this action will leave shadow artifacts that will disrupt the continuity of the CNV network (Figure 1 row 2). This option will be improved in the future — an effective projection resolution algorithm that can more cleanly distinguish projection artifacts from real vessels on a volumetric basis has been demonstrated.6 Another solution is to look at the en face OCTA of the choriocapillaris slab. The projection artifact is less bothersome in the choriocapillaris OCTA, and the CNV is usually associated with a dark halo that sets it off from the normal choroidal circulation (Figure 1 row 3), making it easily recognizable.
It is also useful to look at the color composite en face OCTA that color codes the inner retinal and outer retinal CNV circulations (Figure 1 row 4). This composite view usually allows the CNV to be unambiguously distinguished from the inner retinal vessels by covering up the problem with projection artifacts. Finally, the color cross-sectional OCTA (Figure 1 bottom) is useful in determining if the CNV is below the RPE (type 1) or above the RPE (type 2).
Other excellent uses
OCTA is useful in identifying the full extent of CNV, which is often difficult to see clearly with FA due to obstruction by RPE or hemorrhage. In fact, it is now common to see CNV on OCTA but not FA (no dye leakage) and structural OCT (no fluid space or retinal thickening). These non-exudative CNV are best followed on OCTA.7
OCT is also useful in assessing the effect of anti-VEGF treatment.8-11 On OCTA, the CNV vessel area can be seen to decrease rapidly in the first two weeks after effective anti-VEGF treatment, and fluid re-accumulation tends to be preceded by CNV recrudescence in the form of increasing vessel area.12
Diabetic retinopathy
In diabetic retinopathy, OCTA is most useful in assessing macular ischemia by detecting areas of capillary dropout and retinal neovascularization.13,14 Dividing the macular circulation into superficial and deep plexuses can improve the visualization of capillary dropout, which can be in different areas in the two plexuses (Figure 2). Microaneurysms and dilated vessels can be identified in the deep plexus, and can be associated with edema.15 High contrast of OCTA facilitates evaluation of the foveal avascular zone irregularity, best done using the total inner retinal slab or the superficial slab, which agree well with each other. Three distinct retinal plexuses — superficial, intermediate and deep — can be identified using an advanced projection-resolved OCTA algorithm and may be useful in diabetic retinopathy evaluation in the future.6 Retinal neovascularization can be identified unambiguously as blood vessels in the vitreous slab (Figure 3).
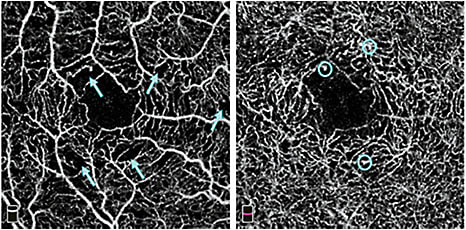
Figure 2. En face retinal OCTA (3×3 mm) in an eye with nonproliferative diabetic retinopathy. Left: superficial plexus in which areas of capillary nonperfusion (blue arrows) can be visualized. Right: deep plexus in which microaneurysms (blue circles) can be seen.
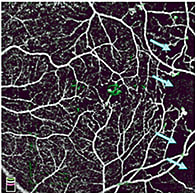
Figure 3. Color composite en face OCTA (6×6 mm) in an eye with proliferative diabetic retinopathy. Inner retinal circulation is in white; vitreous (retinal neovascularization) circulation is in green. There is a small parafoveal retinal neovascularization. Areas of capillary dropout (blue arrows) can be seen temporally.
Other retinal diseases
OCTA has been found to be useful in many retinal diseases.16 It can detect occult CNV in central serous chorioretinopathy,17 pathological myopia, and other conditions. It can detect the lesions in retinal angiomatous proliferation (RAP),18,19 macular telangiectasia (MacTel) 20-22 and polypoidal choroidal vasculopathy (PCV).23,24 For RAP and MacTel, OCTA can visualize the characteristic pathologies of the disease at different levels in the retina and distinguish them from more common type 1 and type 2 CNV. For PCV, OCTA can visualize the branching vascular network with better clarity than ICGA, but sometimes polyps are not visible, in which case ICGA would still be helpful. Retinal capillary nonperfusion can be found in retinal vein occlusion,25,26 radiation retinopathy27 and retinal vasculitis. Choriocapillaris atrophy can be detected in dry AMD28-30 and in inherited retinal dystrophies.29,31 Detection of choriocapillaris nonperfusion was not previously possible with FA/ICGA. However, the clinician should be cognizant that shadowing by drusen and hemorrhage could also lead to apparent defects in choroidal flow on OCTA and must be distinguished from true nonperfusion.
Conclusions
OCTA is a novel imaging technology that has already found wide utility in retinal diseases. It is safer, faster and cheaper than conventional FA and therefore more suitable for routine screening and monitoring.
Although OCTA cannot detect dye leakage and staining, for most diseases the effect of leakage can already be adequately assessed on structural OCT, which can be obtained from the same OCT/OCTA scan. A significant limitation at the present is the relatively small 3- to 6-mm area over which high quality OCTA could be obtained. The field of view will increase as montaging capability is improved and ultra high-speed OCT technology is brought from the laboratory and into the clinical marketplace. But current OCTA technology already offers excellent imaging of the macular and optic disc regions, where most clinically significant pathologies reside. OM
*Casey Eye Institute, Oregon Health & Science University, www.COOLLab.net
References
1. Huang D, Swanson EA, Lin CP, et al. Optical coherence tomography. Science. 1991;254:1178-1181.
2. Jia Y, Tan O, Tokayer J, et al. Split-spectrum amplitude-decorrelation angiography with optical coherence tomography. Opt Express. 2012;20:4710-4725.
3. Gao SS, Liu G, Huang D, Jia Y. Optimization of the split-spectrum amplitude-decorrelation angiography algorithm on a spectral optical coherence tomography system. Opt Lett. 2015;40:2305-2308.
4. Jia Y, Bailey ST, Wilson DJ, et al. Quantitative optical coherence tomography angiography of choroidal neovascularization in age-related macular degeneration.Ophthalmology. 2014;121:1435-1444.
5. Liu L, Gao SS, Bailey ST, et al. Automated choroidal neovascularization detection algorithm for optical coherence tomography angiography. Biomed Opt Express. 2015;6:3564-3576.
6. Zhang M, Hwang TS, Campbell JP, et al. Projection-resolved optical coherence tomographic angiography. Biomed Opt Express. 2016;7:816-828.
7. Palejwala NV, Jia Y, Gao SS, et al. Detection of Nonexudative Choroidal Neovascularization in Age-Related Macular Degeneration with Optical Coherence Tomography Angiography. Retina. 2015;35:2204-2211.
8. Lumbroso B, Rispoli M, Savastano MC. Longitudinal Optical Coherence Tomography-Angiography Study of Type 2 Naive Choroidal Neovascularization Early Response after Treatment. Retina. 2015;35:2242-2251.
9. Spaide RF. Optical Coherence Tomography Angiography Signs of Vascular Abnormalization With Antiangiogenic Therapy for Choroidal Neovascularization. Am J Ophthalmol. 2015;160:6-16.
10. de Carlo TE, Bonini Filho MA, Chin AT, et al. Spectral-domain optical coherence tomography angiography of choroidal neovascularization. Ophthalmology. 2015;122:1228-1238.
11. Kuehlewein L, Bansal M, Lenis TL, et al. Optical Coherence Tomography Angiography of Type 1 Neovascularization in Age-Related Macular Degeneration. Am J Ophthalmol. 2015;160:739-748 e732.
12. Huang D, Jia Y, Rispoli M, Tan O, Lumbroso B. Optical Coherence Tomography Angiography of Time Course of Choroidal Neovascularization in Response to Anti-Angiogenic Treatment. Retina. 2015;35:2260-2264.
13. Hwang TS, Jia Y, Gao SS, et al. Optical Coherence Tomography Angiography Features of Diabetic Retinopathy. Retina. 2015;35:2371-2376.
14. Hwang TS, Gao SS, Liu L, et al. Automated Quantification of Capillary Nonperfusion Using Optical Coherence Tomography Angiography in Diabetic Retinopathy. JAMA Ophthalmol. 2016;1-7.
15. Ishibazawa A, Nagaoka T, Takahashi A, et al. Optical Coherence Tomography Angiography in Diabetic Retinopathy: A Prospective Pilot Study. Am J Ophthalmol. 2015;160:35-44 e31.
16. Huang D, Lumbroso B, Jia Y, Waheed NK (eds). Optical Coherence Tomography Angiography of the Eye. Thorofare, NJ, USA: SLACK Incorporated; 2016.
17. McClintic SM, Jia Y, Huang D, Bailey ST. Optical Coherence Tomographic Angiography of Choroidal Neovascularization Associated With Central Serous Chorioretinopathy. JAMA Ophthalmol. 2015;133:1212-1214.
18. Kuehlewein L, Dansingani KK, de Carlo TE, et al. Optical Coherence Tomography Angiography of Type 3 Neovascularization Secondary to Age-Related Macular Degeneration. Retina. 2015;35:2229-2235.
19. Dansingani KK, Naysan J, Freund KB. En face OCT angiography demonstrates flow in early type 3 neovascularization (retinal angiomatous proliferation). Eye (Lond). 2015;29:703-706.
20. Zeimer M, Gutfleisch M, Heimes B, et al. Association between Changes in Macular Vasculature in Optical Coherence Tomography- and Fluorescein- Angiography and Distribution of Macular Pigment in Type 2 Idiopathic Macular Telangiectasia. Retina. 2015;35:2307-2316.
21. Gaudric A, Krivosic V, Tadayoni R. Outer Retina Capillary Invasion and Ellipsoid Zone Loss in Macular Telangiectasia Type 2 Imaged by Optical Coherence Tomography Angiography. Retina. 2015;35:2300-2306.
22. Spaide RF, Klancnik JM, Jr., Cooney MJ, et al. Volume-Rendering Optical Coherence Tomography Angiography of Macular Telangiectasia Type 2. Ophthalmology. 2015;122:2261-2269.
23. Kim JY, Kwon OW, Oh HS, Kim SH, You YS. Optical coherence tomography angiography in patients with polypoidal choroidal vasculopathy. Graefes Arch Clin Exp Ophthalmol. 2015.
24. Inoue M, Balaratnasingam C, Freund KB. Optical Coherence Tomography Angiography of Polypoidal Choroidal Vasculopathy and Polypoidal Choroidal Neovascularization. Retina. 2015;35:2265-2274.
25. Rispoli M, Savastano MC, Lumbroso B. Capillary Network Anomalies in Branch Retinal Vein Occlusion on Optical Coherence Tomography Angiography. Retina. 2015;35:2332-2338.
26. Nobre Cardoso J, Keane PA, Sim DA, et al. Systematic Evaluation of Optical Coherence Tomography Angiography in Retinal Vein Occlusion. Am J Ophthalmol. 2015.
27. Shields CL, Say EA, Samara WA, Khoo CT, Mashayekhi A, Shields JA. Optical coherence tomography angiography of the macula after plaque radiotherapy of choroidal melanoma: Comparison of Irradiated Versus Nonirradiated Eyes in 65 Patients. Retina. 2016.
28. Kim DY, Fingler J, Zawadzki RJ, et al. Optical imaging of the chorioretinal vasculature in the living human eye. Proc Natl Acad Sci U S A. 2013;110:14354-14359.
29. Jia Y, Bailey ST, Hwang TS, et al. Quantitative optical coherence tomography angiography of vascular abnormalities in the living human eye. Proc Natl Acad Sci U S A. 2015;112:E2395-2402.
30. Choi W, Moult EM, Waheed NK, et al. Ultrahigh-Speed, Swept-Source Optical Coherence Tomography Angiography in Nonexudative Age-Related Macular Degeneration with Geographic Atrophy. Ophthalmology. 2015;122:2532-2544.
31. Jain N, Jia Y, Gao SS, et al. Optical Coherence Tomography Angiography in Choroideremia: Correlating Choriocapillaris Loss With Overlying Degeneration. JAMA Ophthalmol. 2016.
About the Authors | |
![]() | Dr. David Huang is the Peterson Professor of Ophthalmology and Professor of Biomedical Engineering at the Oregon Health & Science University. He is a co-inventor of OCT, and more recently he has pioneered anterior segment OCT and OCT angiography. |
![]() | Dr. Yali Jia |
![]() | Dr. Simon S. Gao |
![]() | Dr. Steven T. Bailey |
![]() | Dr. Thomas S. Hwang |
Financial Interest Disclosure | |
Oregon Health & Science University (OHSU), Dr. Jia and Dr. Huang have a significant financial interest in OptoVue, a company that may have a commercial interest in the results of this research and technology. These potential conflicts of interest have been reviewed and managed by OHSU. |