The argument for swept-source OCT
SS-OCT offers longer wavelengths, a faster scanning speed and wider imaging range.
By Jung Min Lee, MD and Sung Chul (Sean) Park, MD
In 1993, the authors of an article in Optics Letters wrote, “We have recently described a new technique for two- or three-dimensional coherent imaging of biological structure([s)] called optical coherence tomography ([OCT)]1… (which) is particularly attractive in ophthalmic application … it may potentially provide for quantitative diagnosis of a variety of ocular diseases.”2
How prescient they were
In OCT’s newest iteration, swept-source OCT (SS-OCT), that 1993 prognostication is more than affirmed.
Sadly for patients, SS-OCT, generally speaking, is still in FDA approval limbo. It has been used for years in other parts of the world. But, when the technology is finally approved, physicians will be prepared, as numerous researchers have worked on an investigative basis with SS-OCT. In what follows, we will discuss how SS-OCT technology can benefit the diagnosis and management of glaucoma.
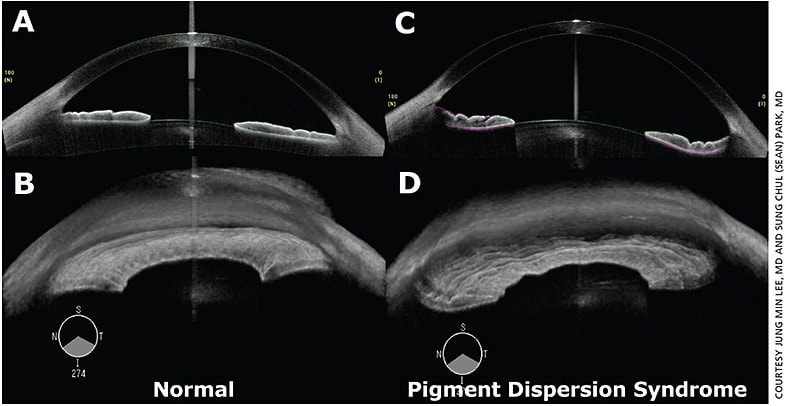
Figure 1. Cross-sectional and 3D images of anterior segment swept-source OCT: (A and B) normal vs. (C and D) pigment dispersion syndrome with concave iris configuration.
An ongoing revolution
The OCT technology family has revolutionized the way clinicians and scientists visualize and analyze structures of the eye, both the anterior and posterior segments. One type, spectral-domain OCT (SD-OCT), offers high axial resolution (approximately 5 µm for SD-OCT vs. 10 µm for time-domain OCT [TD-OCT]) and fast scanning speed (approximately 25,000-55,000 A-scans/s for SD-OCT vs. 100-400 A-scans/s for TD-OCT). SD-OCT also provides reduced signal-to-noise ratio, 3D imaging capability and eye tracking. With regards to glaucoma, these lead to increased reproducibility and more accurate glaucoma progression analysis.3 SS-OCT, which is the most recent evolution of OCT, can further benefit the management of glaucoma because it excels in certain areas while being comparable to other types of OCT.
The argument for swept source
SD-OCT and SS-OCT detect A-scans in the Fourier domain. But, while SD-OCT uses a spectrometer and camera, swept source uses a frequency swept-light source and a high-speed detector to identify the interference signal as a function of time.4
Because the average wavelength of the SS light source is longer than the wavelength of SD-OCT, SS-OCT can reveal more deep-seated tissue structures with less light scattering by lens opacities and less shadowing artifacts from blood vessels or retinal pigment epithelium. Also, SS-OCT’s imaging range is wider and the scanning speed is faster than in SD-OCT, reducing the negative effect of eye movements on OCT image quality.
Anterior segment
Ultrasound biomicroscopy and anterior segment OCT (AS-OCT) are anterior segment imaging techniques that provide objective information of iridocorneal angles, as opposed to gonioscopy’s subjective findings. Although AS-OCT cannot expose the structures behind the iris, it is a non-contact test and has better image resolution compared to ultrasound biomicroscopy.
The Visante AS-OCT (Carl Zeiss Meditec), which is a TD-OCT using a wavelength of 1310 nm, is the only FDA-approved AS-OCT that is designed specifically for imaging the anterior segment. Several retinal SD-OCT devices allow imaging of the anterior segment with higher image resolution, but they provide limited visualization of the angles due to a short wavelength (830 to 870 nm).
Anterior segment SS-OCT complements the shortcomings of TD-OCT and SD-OCTs. Its faster scanning speed and longer wavelength allows for high-resolution, deep-penetration images of the entire anterior segment (radial cross-sectional scans throughout the 360-degree angle circumference) in a few seconds. Studies show an SS-OCT provides reproducible measurements for assessing the risk for angle closure and evaluating longitudinal changes before and after laser iridotomy and peripheral iridoplasty in patients with occludable narrow angles.5-7
The all-important RNFL
Structural changes in the retinal nerve fiber layer (RNFL) are diagnostically significant in glaucoma. These structural changes are associated with functional visual field loss, which can be demonstrated with automated perimetry. However, in glaucoma’s early stages, current technology can detect structural changes in patients without functional visual field loss.8-10 Therefore, the ability to objectively identify and monitor structural changes to the RNFL is essential to the early diagnosis and treatment of glaucoma.
The glaucoma diagnostic capability of RNFL thickness analysis of SS-OCT is similar to that of SD-OCT, a long-approved technology. A recent study by Yang et al. measured the circumpapillary RNFL thickness in glaucomatous and healthy eyes using SS-OCT (DRI-OCT-1 Atlantis, Topcon) and SD-OCT (Spectralis, Heidelberg Engineering GmbH) to compare the glaucoma diagnostic capability of the two OCT machines.11 They found that the area under the ROC curve was similar between SS-OCT and SD-OCT (0.89 and 0.90, respectively).
Peripapillary and macular area
SS-OCT has a large scan window (width of 12 mm and a depth of 8 mm), which enables imaging of the peripapillary retina and macular area in a single scan. DRI-OCT-1 Atlantis can perform serial wide-angle scans covering a 12-mm x 9-mm area of the posterior pole, including the optic nerve head (ONH) and macula. Thickness maps for the RNFL, ganglion cell inner plexiform layer (GCIPL) and ganglion cell complex (GCC) — RNFL and GCIPL — can be created based on these wide-angle posterior pole scans. These thickness maps are useful in detecting and monitoring wedge-shaped RNFL defects and thinning of the macular GCIPL or GCC in glaucoma. In Yang et al’s report, the glaucoma diagnostic capability of SS-OCT’s wide-angle scan (area under the ROC curve = 0.88) was similar to those of SS-OCT’s and SD-OCT’s circumpapillary RNFL scans.11 Combining SS-OCT’s wide-angle scan and the circumpapillary RNFL scan could make the blended glaucoma diagnostic capability greater than that of either one.
SS-OCT’s larger scan window enables better visualization of the posterior segment of highly myopic eyes.12,13 Highly myopic eyes present a challenge when using SD-OCT because the images are often partially inverted or folded. This is due to the limits of the scan window. But SS-OCT, in a single scan, can image and evaluate the peripapillary sclera and its relationship with the ONH along with the macular area.
Lamina cribrosa
Advancements in imaging as well as studies of the human lamina cribrosa in vivo point to this meshwork on the optic nerve head as the principal site of glaucomatous damage to retinal ganglion cell axons.14-21 The lamina cribrosa provides both structural and metabolic support for the axons while creating a barrier between the high-pressure intraocular environment and the low-pressure retrobulbar space.22
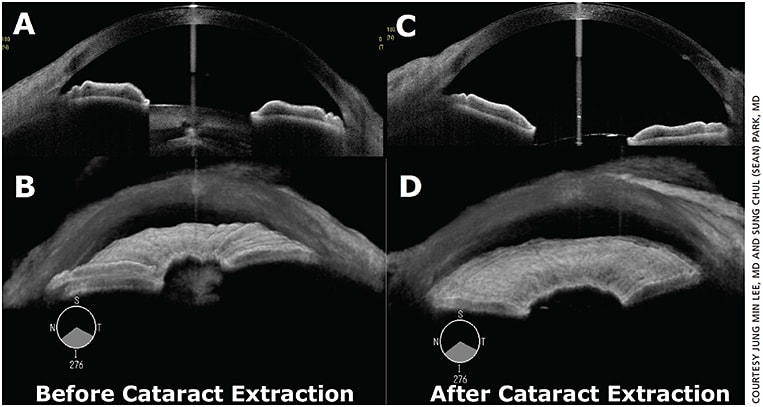
Figure 2. Cross-sectional and 3D images of anterior segment swept-source OCT: (A and B) before vs. (C and D) after cataract extraction with wider iridocorneal angles.
By using an enhanced-depth imaging (EDI) method of SD-OCT to acquire in-depth images of the lamina cribrosa, researchers reinforced earlier histopathological findings that thinning and posterior displacement of the lamina cribrosa were associated with glaucoma.15,16,18,23-25 Focal lamina cribrosa defects and reversal of the posterior lamina cribrosa displacement after intraocular pressure reduction were also demonstrated in glaucomatous eyes using this method.17,19
SS-OCT has also been used to investigate in vivo human lamina cribrosa structures. Takayama et al. detected full-thickness focal lamina cribrosa defects in eyes with glaucoma. They found SS-OCT useful in distinguishing between actual lamina cribrosa defects and artifacts due to vascular shadowing.14 Kwun et al. measured the laminar thickness using SS-OCT and found the lamina cribrosa was thinner in normal-looking fellow eyes of normal-tension glaucoma patients than in the eyes of normal subjects.26 Another study compared SS-OCT and the EDI method of SD-OCT and each’s ability to identify the posterior borders of the sclera and lamina cribrosa. The results showed that SS-OCT better visualized the sclera’s posterior border, but it showed similar visualization of the posterior border of the lamina cribrosa compared to EDI.27
Sclera
IOP affects more than just the lamina cribrosa, including the ONH and peripapillary sclera, which act as a connected system.28 In particular, the sclera acts as the main load-bearing tissue within the eye, and deformations of the sclera are transmitted to ONH tissues. The peripapillary sclera’s stiffness and thickness influence the strain and biomechanical response of the lamina cribrosa to elevated IOP.29 In myopic eyes, scleral thinning and collagen composition changes in the sclera affect the IOP-associated behavior of these eyes.
Several studies have looked at the sclera using SS-OCT in highly myopic eyes — the longer wavelength of SS-OCT allows for deeper tissue penetration compared to SD-OCT. One study measured the subfoveal scleral thickness in 68.4% of subjects.12 The subfoveal scleral thickness was significantly thinner in the normal-tension glaucoma group (496.55 ± 115.20 µm) than in the primary open-angle glaucoma group (670.84 ± 160.60 µm), suggesting that the mechanical properties of the sclera, in addition to IOP, may be related to the pathogenesis of glaucoma. In another study, the entire scleral layer was visualized in 57% of 488 highly myopic eyes with a mean axial length of 29.9 ± 2.0 mm.30
Future implications
SS-OCT provides deeper tissue penetration with a faster scanning speed and wider imaging field compared to SD-OCT — important benefits to glaucoma management. Studies have demonstrated SS-OCT’s ability to evaluate the entire iridocorneal angles within a few seconds in eyes with narrow angles, measure the circumpapillary RNFL thickness reliably, detect focal lamina cribrosa defects in glaucoma, measure the scleral thickness and capture the optic disc and the macula in a single scan.
SS-OCT shows promise in further enhancing the imaging capability of the anterior and posterior segments and identifying new structural parameters for glaucoma diagnosis and monitoring. New SS-OCT devices that can image the entire eye (cornea, iridocorneal angles, vitreous, retina, choroid and sclera) may be developed in the near future. OM
REFERENCES
1. Huang D, Swanson EA, Lin CP, et al. Optical coherence tomography. Science. 1991;254:1178-1181.
2. Swanson EA, Izatt JA, Hee MR, et al. In vivo retinal imaging by optical coherence tomography. Opt Lett. 1993;18:1864-1866.
3. Leung CK, Cheung CY, Weinreb RN, et al. Evaluation of retinal nerve fiber layer progression in glaucoma: a study on optical coherence tomography guided progression analysis. Invest Ophthalmol Vis Sci 2010;51:217-222.
4. Mrejen S, Spaide RF. Optical coherence tomography: imaging of the choroid and beyond. Surv Ophthalmol. 2013;58:387-429.
5. Liu S, Yu M, Ye C, Lam DS, Leung CK. Anterior chamber angle imaging with swept-source optical coherence tomography: An investigation on variability of angle measurement. Invest Ophthalmol Vis Sci. 2011;52:8598-8603.
6. Mishima K, Tomidokoro A, Suramethakul P, Mataki N, Kurita N, Mayama C, Araie M. Iridotrabecular contact observed using anterior segment three-dimensional OCT in eyes with a shallow peripheral anterior chamber. Invest Ophthalmol Vis Sci. 2013;54:4628-635.
7. Ho, SW, Baskaran M, Zheng C, Tun TA, Perera SA, Narayanaswamy AK, Friedman DS, Aung T. Swept source optical coherence tomography measurement of the iris-trabecular contact (ITC) index: a new parameter for angle closure. Arch Clin Exp Ophthalmol. 2013;251:1205-1211.
8. Quigley HA, Addicks EM, Green WR. Optic nerve damage in human glaucoma, III: quantitative correlation of nerve fiber loss and visual field defect in glaucoma, ischemic neuropathy, disc edema, and toxic neuropathy. Arch Ophthalmol. 1982;100:135-146.
9. Harwerth RS, Carter-Dawson L, Shen F, Smith EL 3rd, Crawford ML. Ganglion cell losses underlying visual field defects from experimental glaucoma. Invest Ophthalmol Vis Sci. 1999;40:2242-2250.
10. Medeiros FA1, Lisboa R, Weinreb RN, Liebmann JM, Girkin C, Zangwill LM. Retinal ganglion cell count estimates associated with early development of visual field defects in glaucoma. Ophthalmology. 2013;120:736-744.
11. Yang, Z, Tatham AJ, Zangwill LM, Weinreb RN, Zhang C, Medeiros FA. Diagnostic ability of retinal nerve fiber layer imaging by swept-source optical coherence tomography in glaucoma. Am J Ophthalmol. 2015;159:193-201.
12. Park HY, Lee NY, Choi JA, Park CK. Measurement of scleral thickness using swept-source optical coherence tomography in patients with open-angle glaucoma and myopia. Am J Ophthalmol. 2014;157:876-884.
13. Lim LS, Cheung G, Lee SY. Comparison of spectral domain and swept-source optical coherence tomography in pathological myopia. Eye. 2014;28:488-491.
14. Takayama K, Hangai M, Kimura Y, et al. Three-dimensional imaging of lamina cribrosa defects in glaucoma using swept-source optical coherence tomography. Invest Ophthalmol Vis Sci. 2013;54:4798-807.
15. Lee EJ, Kim TW, Weinreb RN, Park KH, Kim SH, Kim DM. Visualization of the lamina cribrosa using enhanced depth imaging spectral-domain optical coherence tomography. Am J Ophthalmol. 2011;152:87-95.
16. Park, SC, De Moraes CG, Ten CC, Tello C, Liebmann JM, Ritch R. Enhanced depth imaging optical coherence tomography of deep optic nerve complex structures in glaucoma. Ophthalmology. 2012;119:3-9.
17. Kiumehr S, Park SC, Syril D, Teng CC, Tello C, Liebmann JM, Ritch R. In vivo evaluation of focal lamina cribrosa defects in glaucoma. Arch Ophthalmol. 2012;130:552-559.
18. Park HY, Jeon SH, Park CK. Enhanced depth imaging detects lamina cribrosa thickness differences in normal tension glaucoma and primary open-angle glaucoma. Ophthalmology. 2012;119:10-20.
19. Lee EJ, Kim TW, Weinreb RN. Reversal of lamina cribrosa displacement and thickness after trabeculectomy in glaucoma. Ophthalmology. 2012;119:1359-1366.
20. Minckler DS, Bunt AH, Johanson GW. Orthograde and retrograde axoplasmic transport during acute ocular hypertension in the monkey. Invest Ophthalmol Vis Sci. 1977;16:426-441.
21. Quigley HA, Addicks EM, Green WR, Maumenee AE. Optic nerve damage in human glaucoma, II: the site of injury and susceptibility to damage. Arch Ophthalmol. 1981;99:635-649.
22. Anderson DR. Ultrastructure of human and monkey lamina cribrosa and optic nerve head. Arch Ophthalmol. 1969;82:800-814.
23. Spaide RF, Koizumi H, Pozzoni MC. Enhanced depth imaging spectral-domain optical coherence tomography. Am J Ophthalmol. 2008;146:496-500.
24. Furlanetto RL, Park SC, Damle UJ, Sieminski SF, Kung Y, Siegal N, Liebmann JM, Ritch R. Posterior displacement of the lamina cribrosa in glaucoma: in vivo interindividual and intereye comparisons. Invest Ophthalmol Vis Sci. 2013;54:4836-4842.
25. Park SC, Brumm J, Furlanetto RL, Netto C, Liu Y, Tello C, Liebmann JM, Ritch R. Lamina cribrosa depth in different stages of glaucoma. Invest Ophthalmol Vis Sci. 2015;56:2059-2064.
26. Kwun Y, Han JC, Kee C. Comparison of lamina cribrosa thickness in normal tension glaucoma patients with unilateral visual field defect. Am J Ophthalmol. 2015;159:512-518.
27. Park HY, Shin HY, Park CK. Imaging the posterior segment of the eye using swept-source optical coherence tomography in myopic glaucoma eyes: comparison with enhanced-depth imaging. Am J Ophthalmol. 2014;157:550-557.
28. Sigal IA, Yang H, Roberts MD, et al. IOP-induced lamina cribrosa deformation and scleral canal expansion: independent or related? Invest Ophthalmol Vis Sci. 2011;52:9023-9032.
29. Eilaghi A, Flanagan JG, Simmons CA, Ethier CR. Effects of scleral stiffness properties on optic nerve head biomechanics. Ann Biomed Eng. 2010;38:1586-1592.
30. Ohno-Matsui K, Akiba M, Modegi T, Tomita M, Ishibashi T, Tokoro T, Moriyama M. Association between shape of sclera and myopic retinochoroidal lesions in patients with pathologic myopia. Invest Ophthalmol Vis Sci. 2012;53:6046-6061.
About the Author | |
![]() | Jung Min Lee, MD, is a clinical glaucoma fellow at Manhattan Eye, Ear and Throat Hospital, New York, N.Y. Her e-mail is jungminlee@gmail.com. |
![]() | Sung Chul (Sean) Park, MD, is the director of Glaucoma Research and Glaucoma Fellowship Program at Manhattan Eye, Ear and Throat Hospital, New York, N.Y. His e-mail is sungchulpark1225@gmail.com. |
Dr. Park and Dr. Lee have no relevant financial disclosures. |