We’ve come a-ways since the slit lamp
Advances in anterior segment imaging allow access to more information, leading to more precise patient care.
By Martin L. Fox, MD, FACS
When I finished my training, residency and corneal fellowship, the available technology for evaluating the anterior segment was limited to the slit lamp and lenses, clinical acumen and a modicum of intuition. Since then, anterior segment imaging has evolved to the point that the general ophthalmologist has access to modalities that can precisely image ocular anatomy allowing for remarkable progress in precise diagnostic and surgical planning. Among other functions, the technology helps to: increase knowledge of a patient’s cornea anatomy following laser vision correction; precisely evaluate the chamber angle and anterior chamber depth in preparation for phakic IOL surgery; and determine accurate corneal power information to assist in IOL power selection and variety prior to cataract surgery.
Rotating Scheimpflug imaging, anterior segment optical coherence tomography (OCT), advanced ultrasound biomicroscopy (UBM) and high-resolution MRI now provide valuable information on the anterior segment allowing for evaluation of the characteristics of angle structures, ciliary body, crystalline lens and the cornea.
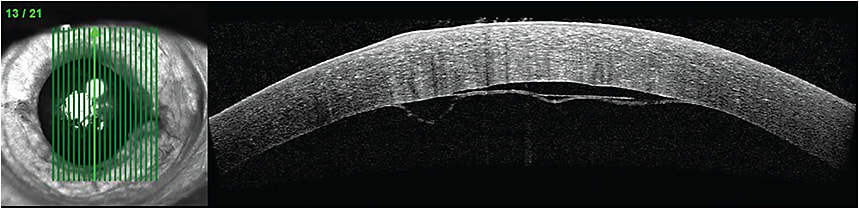
A spectral domain OCT image of a Descemet’s membrane detachment.
COURTESY TIMOTHY J. BENNETT, CRA, FOPS, PENN STATE HERSHEY EYE CENTER
Rotating Scheimpflug imaging
The Scheimpflug principle has been long appreciated for its ability to take images of the anterior segment via a rotating camera that captures information on structures not parallel to the plane of focus. The most notable Pentacam instrument (Oculus) can take up to 50 slit images of the anterior segment in less than two seconds allowing for calculation of corneal topography, anterior and posterior corneal anatomical and power information and anterior chamber depth. A newer version, PentacamHR, includes phakic intraocular lens (IOL) software to simulate the position of a proposed lens. It also allows for calculation of a corrected intraocular pressure based on corneal thickness.
OCT
A well-established imaging technique, OCT is based on a low coherence interferometry, making use of near-spectrum infrared light to capture micrometer resolution and three-dimensional images. The nature of this long wavelength light allows penetration into the light-scattering ocular medium. Developed in 1990 by Naohiro Tanna and further defined by Huang et al. in 1991, OCT has become the pre-eminent modality in anterior segment tissue imaging.1,2
The OCT principal works very much like ultrasound, using light emission and reflection rather than sound waves. The process of coherence interferometry calculates time delay and intensity of back-scattered light against a known reference path length and time allowing for the generation of clear 3D images. The reflectivity profile (A-scan) contains information about spacial dimensions of tissues of interest that have unique reflectivity characteristics. Time domain OCT allows for maximum resolution of about 2 to 10 microns, equating to a 10- to 50-fold greater resolution than ultrasound.3
OCT technology was initially applied in retinal diagnostics while the anterior segment versions, designed later, have made use of longer wavelengths of light (1310 nm vs. 820 nm) allowing for greater tissue penetration and better visualization of structures that characteristically scatter light.
Visualization of retro-iris, ciliary body and sulcus is now possible. An example of time domain OCT technology, the Visante OCT (Carl Zeiss Meditec), works at 1310 nm and provides non-contact imaging of the anterior segment and anterior chamber angle with a rate of up to 2048 A-scans per second. Its several anterior segment-scanning protocols include the anterior segment single scan and high-resolution raw scan.
More recently available, spectral domain OCT (SD-OCT) systems have demonstrated the potential for much more rapid axial scanning.4 SD-OCT works by analyzing the reflected signal as a function of wavelength of light and can allow for scanning rates that are many orders of magnitude higher. The Spectralis system (Heidelberg Engineering), for example, measures multiple wavelengths of reflected light across a spectrum and is 100 times faster than traditional time domain-based systems, acquiring 40,000 A-scans per second for significantly higher resolution. Also, its associated eye tracker system prevents motion artifact and incorporates confocal scanning laser technology to allow for additional high contrast fundus imagery.
Four SD-OCT products are on the market:
Cirrus (Carl Zeiss Meditec), RTVue-100 (Optovue Inc.), Spectralis system (Heidelberg Engineering) and Topcon 3D-OCT 20009 (Topcon Corp).
(Optos’ anterior segment OCT exists via retrofitting its OCT SLO with an AC cornea lens. The system provides high-resolution spectral imaging.)
High-resolution MRI
UBM and OCT possess some distinct disadvantages when compared to HR MRI. With UBM, an image-impeding gel must be applied to the eye, and penetration depth is limited. Scheimpflug imaging and OCT are limited by the iris, which obscures ciliary muscle, zonular fibers and lens periphery. But high resolution MRI has become a useful tool in ophthalmic imaging because it views the entire globe. This instrument has allowed for a better understanding of mechanisms of accommodation, aiding in the development and investigation of new IOL designs.5
The future of anterior segment OCT imaging
In the very near future advanced OCT imaging technology will become available. These devices include higher resolution OCT and ultra high-speed swept OCT, allowing for axial image enlargement and adaptive optics OCT, incorporating the mechanism to neutralize inherent focusing errors in the eye under examination for better image clarity. OM
REFERENCES
1. Tanno N, Ichikawa T, Saeki A. Lightwave Reflection Measurement. Japanese patent #2010042, 1990.
2. Huang D, Swanson EA, Lin CP, et al. Optical Coherence Tomography. Science. 1991;254:1178-1781.
3. Fercher AF. Ophthalmic Interferometry. Proceedings of the International Conference on Optics and Life Sciences, Garmisch-Partenkirchen, Germany 12-16 August 1990.
4. Major JC Jr, Wykoff CC, Mariani AF, et al. Comparison of spectral-domain and time-domain optical coherence tomography in the detection of neovascular age-related macular degenerationactivity. Retina 2014. Jan;34:48-54.
5. Advanced Physiologic Optics Laboratory. www.cvs.rochester.edu/yoonlab/research. Accessed Aug. 20, 2015.
About the Author | |
![]() | Dr. Fox is medical director at the Cornea and Refractive Surgery Practice of New York and at Clarity Refractive Services of West Orange, N.J. E-mail him at foxmd@laserfox.com or by phone 917-207-3147. His website is www.laserfox.com. |