A new view for an old disease
SSADA software, coupled with OCT angiography shows potential in diagnosing, monitoring glaucoma.
By David Huang, MD, PhD, et al.*
Measuring the thicknesses of both the peripapillary retinal nerve fiber layer (RNFL) and ganglion cell complex (GCC) with spectral-domain optical coherence tomography (SD-OCT) helps with identifying and monitoring structural damage caused by glaucoma. While these methods show promise as objective quantifiable measures for glaucoma assessment, they have limited sensitivity for detecting early glaucoma and only moderate correlation with visual field (VF) loss.1-9 Vascular defects could be another objective method used to evaluate glaucoma.
A number of studies show glaucoma (first listed by Hippocrates among infirmities of the aged) could be associated with vascular dysfunction.10-16 Laser Doppler flowmetry and laser speckle flowgraphy (LSFG) have shown a reduction in optic nerve head (ONH) and peripapillary blood flow dynamics in glaucoma.15,17-22 However, measurements using these methods vary too much between normal subjects, so it is difficult to distinguish glaucoma from normal variation on an individual basis.
OCT angiography could be the answer to these issues.
A new algorithm, and no dye
OCT angiography provides detailed 3-dimensional images of retinal circulation down to the capillary level. It detects flow by measuring the variability of the OCT signal over consecutive B-frames at the same location. Because it uses intrinsic motion contrast, no injection of extrinsic dye is needed.
OCT angiography has been investigated for nearly a decade. But until recently, it was not practical for clinical applications because it required many repeat scans at the same location and took too long at the speed of commercially available OCT systems. A novel software algorithm, called split-spectrum amplitude-decorrelation angiography (SSADA) and developed by Dr. Huang’s research group, solved the speed problem. SSADA splits each OCT image into up to 11 spectral (wavelength) divisions. Because each spectral channel has an independent speckle pattern, recombining the speckle decorrelation signal (a metric for variation) greatly improves the signal-to-noise ratio of flow detection.
This highly efficient algorithm can provide high quality angiograms using as few as two B-frames at each location. The SSADA software for the SD-OCT platform is commercially available internationally (Angiovue, Optovue). It is pending 510k approval by the FDA.
In an initial study using the SSADA software on a swept-source OCT, Dr. Huang’s group quantified optic disc perfusion and showed decreased disc perfusion in glaucoma.13 In a subsequent pilot study, the optic disc region was imaged by 3mm x 3mm scans using the commercial Angiovue software to evaluate the peripapillary retinal perfusion and vessel density. This was the first attempt to use OCT angiography for quantitative evaluation of the peripapillary retinal vasculature in glaucoma.
In this pilot study, researchers looked at 12 glaucomatous eyes (nine with perimetric glaucoma and three with pre-perimetric glaucoma) and 12 age-matched normal eyes. They obtained OCT angiograms of the peripapillary retina and compared perfusion indices (flow index and vessel density) between normal and glaucoma participants. Correlation between these perfusion indices and more conventional measures of glaucoma (VF and structural OCT) were also investigated.
Methods and data acquisition
One eye from each participant in the Functional and Structural Optical Coherence Tomography for Glaucoma study was scanned and analyzed. Researchers performed VF tests with the Humphrey Field Analyzer II (Zeiss). They obtained an en face OCT angiogram of the retinal circulation by the maximum flow (decorrelation value) projection from the inner limiting layer to retinal epithelial pigment.
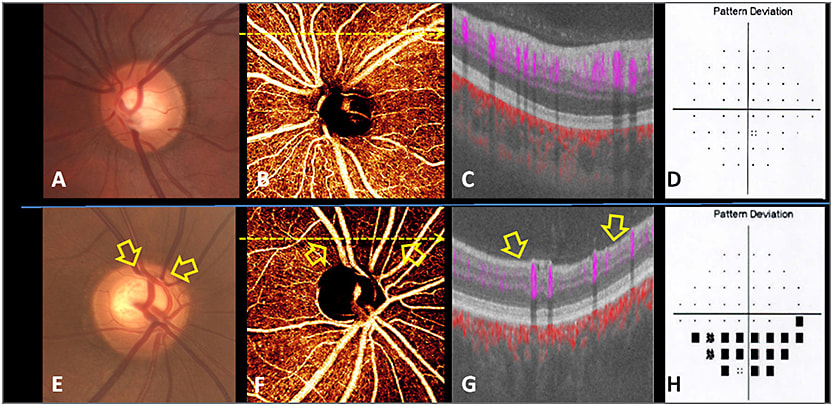
Figure 1. Shows images of normal and PG eyes. Disc photographs (A, E) show pink rim in the normal eye (A) and superior notch in the glaucomatous eye (E). In the normal eye, a dense microvascular network around the disc was visible on OCT angiography (B). This network was visibly attenuated in glaucomatous eyes, with focal capillary dropout (arrow) detected (F). OCT cross-sections (C, G) overlaying retinal flow (purple) on OCT reflectance (gray scale) show a perfusion defect associated with nerve fiber layer thinning (arrow). The VF pattern deviation maps (D, H) show inferior depression matching the location of perfusion defect in the glaucomatous eye (H).
The peripapillary region was defined as a 700-µm wide elliptical annulus extending from the optic disc boundary. The peripapillary flow index, which measures the area of large vessels and both the area and velocity of retinal microvasculature, was defined as the average decorrelation value on the en face retinal angiogram.13 The vessel density was defined as the percentage area occupied by the large vessels and microvasculature in the peripapillary region. The nerve fiber layer thickness was averaged from a circular sampling profile of 3.4-mm diameter centered on the disc.
Within-visit repeatability of the flow index and vessel density was calculated with two sets of scans performed sequentially within a single visit. Variability was assessed by the coefficient of variation (CV).
Peripapillary retinal flow index and vessel density comparisons
Both the flow index and vessel density in the glaucoma group were statistically lower than that of the normal group. They are in agreement with a previous swept-source OCT study; earlier LDF and LSFG studies show decreased peripapillary and ONH blood flow in glaucoma.15,17-22
The advantage of OCT angiography is that there is very little variation in vessel density within the normal population, therefore even small amounts of glaucomatous damage can be detected with high confidence. OCT angiography also offers high within-visit repeatability, high between-visit reproducibility and inter-operator reproducibility, a marked improvement compared to other noninvasive techniques such as LDF and LSFG. OCT angiography is more viable for use in a clinical application. The area under the receiver operating curve (AROC) for differentiating normal and glaucoma participants was 0.892 for flow index and 0.938 for vessel density. This may point to a loss in peripapillary retinal perfusion as a potentially reliable diagnostic parameter to differentiate normal participants from those with glaucoma.
Traditional glaucoma diagnostic measurement correlation
In the glaucoma group, Pearson’s correlation coefficient showed that both flow index and vessel density were highly correlated with the VF pattern standard deviation. The findings of moderate correlation between VF indices and RNFL thickness were similar to other studies.1,3,7-9 The glaucoma participants ranged from those with pre-perimetric glaucoma to moderate and severe. The flow index and vessel density demonstrated high correlation with glaucoma stage, which is determined by the glaucoma staging system 2.23
In contrast, glaucoma staging and RNFL thickness showed no significant correlation. These data suggest that blood flow indices measured by OCT may be more meaningful indicators of glaucoma severity than structural measures. The perfusion indices may have potential for staging and monitoring glaucoma.
Summary
High-quality OCT angiograms of the peripapillary retina could be obtained in both normal and glaucoma participants using the SSADA software in a commercially available OCT. Reduction in peripapillary retinal perfusion in eyes with glaucoma could be visualized as focal defects and quantified as flow index and vessel density with high repeatability and reproducibility. The area of reduced perfusion matched the VF defect and NFL loss, but also was present in some eyes with a VF defect before an NFL defect was visible. This would allow for earlier diagnosis of glaucoma.
This tight correlation between peripapillary perfusion and VF suggests OCT angiography has the potential for improving glaucoma monitoring, even in eyes with more advanced disease, where the ability to detect progression based on further structural thinning is limited because of the “floor effect.”
Quantitative OCT angiography has potential usefulness in the diagnosis, staging and monitoring of glaucoma. OM
*Additional authors: Liang Liu, MD, Yali Jia, PhD, Hana L. Takusagawa, MD, Alex D. Pechauer, BS, Beth Edmunds, MD, PhD, Lorinna Lombardi, MD, Ellen Davis, MD, John C. Morrison, MD
REFERENCES
1. Abadia B, Ferreras A, Calvo P, et al. Relationship between spectral-domain optical coherence tomography and standard automated perimetry in healthy and glaucoma patients. Biomed Res Int. 2014;2014:514948.
2. Wu H, de Boer JF, Chen TC. Diagnostic capability of spectral-domain optical coherence tomography for glaucoma. Am J Ophthalmol. 2012;153:815-826 e812.
3. Nilforushan N, Nassiri N, Moghimi S, et al. Structure-function relationships between spectral-domain OCT and standard achromatic perimetry. Invest Ophthalmol Vis Sci. 2012;53:2740-2748.
4. Garas A, Vargha P, Hollo G. Comparison of diagnostic accuracy of the RTVue Fourier-domain OCT and the GDx-VCC/ECC polarimeter to detect glaucoma. Eur J Ophthalmol. 2012;22:45-54.
5. Sung KR, Lee S, Park SB, et al. Twenty-four hour ocular perfusion pressure fluctuation and risk of normal-tension glaucoma progression. Invest Ophthalmol Vis Sci. 2009;50:5266-5274.
6. Chang RT, Knight OJ, Feuer WJ, Budenz DL. Sensitivity and specificity of time-domain versus spectral-domain optical coherence tomography in diagnosing early to moderate glaucoma. Ophthalmology. 2009;116:2294-2299.
7. Mok KH, Lee VW, So KF. Retinal measurement by optical coherence tomography in glaucoma suspects with short-wavelength perimetry abnormalities. J Glaucoma. 2003;12:45-49.
8. El Beltagi TA, Bowd C, Boden C, et al. Retinal nerve fiber layer thickness measured with optical coherence tomography is related to visual function in glaucomatous eyes. Ophthalmology. 2003;110:2185-2191.
9. Williams ZY, Schuman JS, Gamell L, et al. Optical coherence tomography measurement of nerve fiber layer thickness and the likelihood of a visual field defect. Am J Ophthalmol. 2002;134:538-546.
10. Wong TY, Mitchell P. The eye in hypertension. Lancet. Feb 3 2007;369:425-435.
11. Harris A, Rechtman E, Siesky B, et al. The role of optic nerve blood flow in the pathogenesis of glaucoma. Ophthalmol Clin North Am. Sep 2005;18:345-353, v.
12. Bonomi L, Marchini G, Marraffa M, et al. Vascular risk factors for primary open angle glaucoma: the Egna-Neumarkt Study. Ophthalmology. 2000;107:1287-1293.
13. Jia Y, Wei E, Wang X, et al. Optical coherence tomography angiography of optic disc perfusion in glaucoma. Ophthalmology. 2014;121:1322-1332.
14. Flammer J. The vascular concept of glaucoma. Surv Ophthalmol. May 1994;38 Suppl:S3-6.
15. Tobe LA, Harris A, Hussain RM, et al. The role of retrobulbar and retinal circulation on optic nerve head and retinal nerve fibre layer structure in patients with open-angle glaucoma over an 18-month period. Br J Ophthalmol. 2015;99:609-612.
16. Flammer J, Orgul S, Costa VP, et al. The impact of ocular blood flow in glaucoma. Prog Retin Eye Res. 2002;21:359-393.
17. Yokoyama Y, Aizawa N, Chiba N, et al. Significant correlations between optic nerve head microcirculation and visual field defects and nerve fiber layer loss in glaucoma patients with myopic glaucomatous disk. Clin Ophthalmol. 2011;5:1721-1727.
18. Piltz-seymour JR, Grunwald JE, Hariprasad SM, Dupont J. Optic nerve blood flow is diminished in eyes of primary open-angle glaucoma suspects. Am J Ophthalmol. 2001;132:63-69.
19. Michelson G, Langhans MJ, Groh MJ. Perfusion of the juxtapapillary retina and the neuroretinal rim area in primary open angle glaucoma. J Glaucoma.1996;5:91-98.
20. Hamard P, Hamard H, Dufaux J, Quesnot S. Optic nerve head blood flow using a laser Doppler velocimeter and haemorheology in primary open angle glaucoma and normal pressure glaucoma. Br J Ophthalmol. 1994;78:449-453.
21. Nizankowska MH, Jamrozy-Witkowska A, Asejczyk M. Evaluation analysis of blood flow of peripapillary area in glaucoma patients using scanning laser Doppler flowmetry. Klin Oczna. 2002;104:201-206.
22. Sugiyama T, Shibata M, Kojima S, Ikeda T. Optic nerve head blood flow in glaucoma. In: Kubena T, editor. ed Mystery of Glaucoma. New York: InTech; 2011: 207-218.
23. Brusini P, Filacorda S. Enhanced Glaucoma Staging System (GSS 2) for classifying functional damage in glaucoma. J Glaucoma. 2006;15:40-46.
About the Author | |
![]() | David Huang, MD, is the Peterson Professor of Ophthalmology and Professor of Biomedical Engineering at the Oregon Health & Science University. Contact him at davidhuang@alum.mit.edu |