Replacing the dead with the living
The NEI has an audacious goal: to regenerate dead photoreceptors and restore sight robbed by disease.
By Christine Bahls, Executive Editor, and Zack Tertel, Senior Editor
Today, if a patient’s sight is diminished or lost, be it by injury, disease or genetic malfunction, he won’t hear the words ‘sight restoration’ from his physician. Nerve cells in the retina and brain that die are lost forever.
Keep in mind that at least one-third of the brain — tough to study noninvasively — is devoted to transforming what we see, into what we see. For these reasons, the retina and the optic nerve, replete with its 75+ kinds of retinal-located glia and neurons that liaise to transform light into vision, are ground zero for a new National Eye Institute (NEI) project whose primary focus is restoring sight through regenerating and reconnecting nerve cells that have died as a result of disease or injury.1,2
Explaining audacious
Dubbed the Audacious Goals Initiative (AGI), its aim, says Michael A. Steinmetz, PhD, acting director, Division of Extramural Research at the National Eye Institute, is to lay the genetic and technological groundwork to restore sight lost to blinding diseases. Earlier this year, five research teams received grants totaling $3.8 million over five years, to design or adapt technologies whose focus is to image the retina and optic nerve.
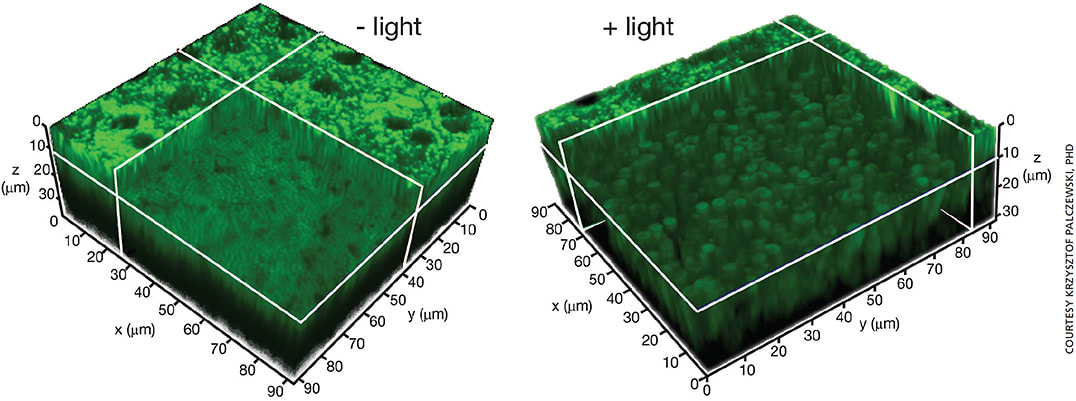
Figure 1. TPM images of mouse retina.
A. 3-D TPM image of the retina and RPE in an intact Abca4-/-Rdh8-/- mouse eye. Image obtained eight hours after exposure to bright light. RPE cells are at the top of the 3-D section, and the photoreceptor cell layer section is shown 10 μm below the RPE.
B. TPM 3-D image of the retina and RPE in an intact Abca4-/-Rdh8-/- mouse eye. Image obtained on day two after exposure to bright light. RPE cells are at the top of the 3D section, and the enlarged, doughnut-shaped photoreceptors are shown 10 μm below the RPE.
AGI investigator Q&A
Q: What was the genesis of the project?
Austin Roorda, PhD, University of California
The initial concepts started while I was on a six-month sabbatical leave in the Netherlands with Professor Johannes deBoer in early 2012. He is an early pioneer in OCT technology and he wanted to use OCT for eye tracking to correct for eye movements.
Krzysztof Palczewski, PhD, Case Western Reserve University, Cleveland
Fluorescence imaging of the retina-RPE interface provides information about its fluorophore content, which reflects both the efficiency of the retinoid cycle and its responses to aging, external stress, genetic manipulations and pharmaceutical treatments. However, these fluorophores, including vitamin A-derived retinoids, which have absorption spectra in ultraviolet range, are not transmitted through the lens of the human eye. The idea of using two-photon excitation was born.
In two-photon imaging, use of long-wavelength and nonlinear excitation beams bypasses the absorption of ultraviolet light by molecules outside its focus, and allows imaging of native tissues with subcellular resolution depths not attainable by other optical modalities. We first discussed two-photon excitation imaging to study biochemical processes that participate in sustaining mammalian vision in a 2004 Journal of Biological Chemistry paper (http://www.ncbi.nlm.nih.gov/pmc/articles/PMC1360214/).
Alfredo Dubra, PhD, Medical College of Wisconsin, Milwaukee
We focus on developing tools to facilitate high-resolution imaging of the living retina. In addition to building instruments, we have replicated them at other institutions, including University College London in the UK, University of Pennsylvania, New York Eye and Ear Infirmary of Mount Sinai, UC San Diego and the National Eye Institute research campus. It became pretty apparent from using this technology in over two thousand patients with many different conditions that although it’s okay as proof of principle, it’s still not ready for mainstream use.
Sheng-Kwei (Victor) Song, PhD, Washington University, St. Louis
Our lab has been working on developing a biomarker of nerve injury. Our imaging method, in mice with optic neuritis, detected, distinguished and quantified various types of damage in the optic nerve that before only happened with postmortem histology. We further demonstrated that water diffusion properties in the optic nerve changed during visual stimulation. The extent of this change was significantly reduced in mice with optic neuritis correlating with impaired visual acuity. Thus, we proposed to develop a diffusion MRI method that would let us simultaneously image the optic nerve function and the pathology.3
When we look at the optic nerve, we can see the effect that diffusion has on changes in the nerve — we can measure simultaneously the pathology and the function of the nerve using diffuse MRI.
David Williams, PhD, the William G. Allyn Professor of Medical Optics, Dean for Research and director of the Center for Visual Science, University of Rochester
Molecular biology is creating exciting new avenues for restoring vision In the blind that we believe will eventually transform clinical ophthalmology, including gene therapeutic, optogenetic, and stem cell approaches. We also believe that a major limitation on progress in vision restoration is the lack of tools to rapidly evaluate successes and failures of various strategies for restoring vision. A major thrust of our AGI project is to develop new tools that can potentially accelerate the development of any vision-restoration approach. My colleagues and I at the University of Rochester have been developing technology for high resolution imaging of the retina for more than two decades. We exploit adaptive optics, a technology originally conceived to improve astronomical imaging, to improve ophthalmoscopic images of the retina to the point where single cells can be resolved in individual layers in the living eye. Our AGI project is a collaboration between our imaging team at Rochester, which consists of Jennifer Hunter, Bill Merigan and myself, and three laboratories at our partnering institutions, each of which have expertise in different approaches to vision restoration. Our partners include Connie Cepko’s laboratory at Harvard Medical School, which brings expertise in gene therapy, Botond Roska’s group at the Fredrich Miescher Institute for Biomedical Research in Basel, Switzerland with expertise in optogenetics, and David Gamm’s laboratory at the University of Wisconsin, which brings expertise in stem cell therapy for vision restoration.
Q: What have you learned from the workshops with the other project investigators?
Dr. Palczewski
The diverse group of investigators is developing new technologies to noninvasively image cells of the eye in unprecedented detail. These new approaches benefit from the synergy between vast knowledge in optics, physiology and biochemistry that sustain visual processes. We hope to apply tools that are already developed by other laboratories to assess potential safety issues when engineering the newly developed instrumentation for applications in humans.
Dr. Williams
One of the most important messages I have taken away is the growing recognition of the importance of nonhuman primate models as a necessary stepping stone to the development of vision restoration methods in humans. Nonhuman primates have an eye and a retinal structure that are very similar to humans, making them extremely valuable in the development of viable approaches that can ultimately be deployed in patients.
Q: What do you hope to learn from your research?
Dr. Dubra
One population that stands to benefit most from gene and stem cell therapies is still beyond reach — those with nystagmus, which is extreme involuntary eye motion. The rapid eye motion prevent us from capturing pictures of the retina at the microscopic scale, so we are proposing a new approach to eye motion compensation that takes advantage of advantage of novel camera and image processing technologies.
Dr. Song
I think our method will see injury regardless of the injury’s origin. We can distinguish axon and myelin injury, for example, from inflammation. Functionally you might not be able to tell what causes the visual impairment. With our method, we could determine whether the visual impairment results from reversible inflammation or irreversible axon loss. This will let physicians stratify therapies and target a specific pathology.
Dr. Williams
The goal is to accelerate the development of each of these approaches to vision restoration by deploying adaptive optics imaging to evaluate whether these approaches can restore or partially restore functional neural circuitry in animal models of retinal degeneration. The key capability we have already developed and are constantly improving is to track individual cells in the living eye over time, most recently using fluorescence imaging that can reveal the neural activity of indiindividual cells in the retina. As one example, we ultimately hope to track individual stem cells implanted in the nonhuman primate retina, designed to replace photoreceptors destroyed by disease, to determine the conditions that might allow them to successfully connect to other neurons in the retina. We also are developing the tools to record optically from retinal ganglions to determine whether these implanted stem cells are capable of generating signals in response to light that can be conveyed to the output neurons of the retina.
Q: How do you see your research ultimately benefiting ophthalmologists, and when?
Dr. Roorda
In the early stages, the use of advanced tools to image structure and function in the retina, like the one we’re developing, will play a crucial role in testing and demonstrating the efficacy of all the promising new treatments for degenerative retinal diseases. In the longer term, these advanced tools may ultimately be used in the clinic to provide sensitive objective assessments of a patient’s retinal health.
Dr. Dubra
For most ophthalmologists, at least five years, maybe longer. But some techniques we have developed in the last three to four years, in particular split-detection imaging, are already being used by people developing gene therapies in University College London and in U Penn. Until we developed this imaging method, it was not possible to identify which patients had enough cone photoreceptors left to benefit from the treatment, as gene therapy assumes you have some cells in the retina that are not working properly and you’re going to repair them.
Dr. Palczewski
The two-photon excitation approach has the potential to both detect early molecular changes in retinoid metabolism that trigger light- and AMD-induced retinal defects, and assess the effectiveness of treatments for these conditions. The instrument could identify and follow abnormal individual biochemical transformations well before pathophysiological transformations can be detected by current methodology.
Our group has reported on the use of a noninvasive two-photon excitation imaging approach to assess the effectiveness of novel treatments for retinal degeneration. These include the sequestration of toxic-free all-transretinal, a systems pharmacology approach to treat retinal degeneration, and rescue of vision by 9-cis-retinoids. Identifying new therapies that prevent degenerative processes leading to blindness could benefit greatly from early detection and more sensitive assays, facilitating a shorter duration of clinical trials).
Dr. Williams
Our hope is that the imaging tools we are developing may eventually be used by the practicing ophthalmologist to track the efficacy of many therapies for retinal disease in their patients, and that our AGI project will ultimately help to deliver a new suite of therapies for retinal degeneration.
The institute feels that regeneration of lost cells, and reconnection to the brain via the optic nerve, is possible. “We are developing a better and better understanding of what normal function is, [including what it] looks like in the retina and the visual system in the brain,” says Dr. Steinmetz, an architect of the initiative. “If you have a good understanding of normal function, you have a chance of grasping what goes wrong in disease.”
This knowledge is due to the eye’s easy accessibility — via drops, microscope, light waves — that has made it a popular model for nervous system research. And, since its inception in 1968, the NEI has invested time and money into basic research of the visual system.
Accessibility alone, however, doesn’t allow a researcher to see if cells are in proper working order. The NEI, explains Dr. Steinmetz, wants new tools made available to clinicians to image the eye “and see not just its structure, but to see how cells in the eyes are functioning.”
A singular design
The AGI’s design is as singular as its purpose. It began in 2012: 500 people submitted ideas to an AGI competition; 10 winning ideas were used to shape the AGI. In April 2014, the NEI issued a request for proposals to develop new ways to image the visual system, and the five grantees were selected through NIH peer review. The groups will gather for periodic workshops with key leaders in various fields to exchange ideas, data, and technology. And, each group will report on its progress to the NEI each year.
“All will benefit if the [design] is implemented properly,” says Jeffrey L. Goldberg, MD, PhD, professor and chair, Department of Ophthalmology, Byers Eye Institute at Stanford University, who co-chaired an AGI workshop on regenerating the optic nerve. “Cross-discipline collaboration can only help to push the science forward.”
Austin Roorda, PhD, associate professor of optometry and vision science, University of California, Berkeley, one of the five, says the workshops have been “great.” While the imaging projects represent a broad range of concepts, he continues, there is a great deal of synergy between them. His project? To design an imaging tool that can show — without distortion — the structure, and function, of human retinas in healthy and diseased states. If successful, his lab’s imaging device will be able to measure the functional activity of single cells in the retina.
Clinical implications
Regeneration of the optic nerve has “profound implications for clinical ophthalmology,” says Dr. Goldberg. But the answer as to how to make that happen remains “rooted” in neuroscience.
So these researchers are seeking answers at the cellular level. Alfredo Dubra, PhD and Joseph Carroll, PhD of the Medical College of Wisconsin are working with Jessica Morgan, PhD and David Brainard, PhD at the University of Pennsylvania on an interesting behavior of individual photoreceptors; they twinkle, and even flicker faster if light is flashed on them. “We want to see if these intensity changes could give us information on the cell’s health. You could think of it as a single-cell optical EKG.”
The hope, says Dr. Dubra, is to replace tedious and often unreliable subjective visual tests that only test a small percentage of our vision with objective ones that provide individual information on thousands of cells.
In the lab of Krzysztof Palczewski, PhD, Case Western Reserve University, Cleveland, the focus is on vitamin A, and more specifically, how retinal diseases affect vitamin A’s capabilities to power photoreceptors. This detective work extends to studying the visual system’s efficiency before pathologic changes are discernible with existing imaging tools. He is developing a two-photon ophthalmoscope for imaging and testing.
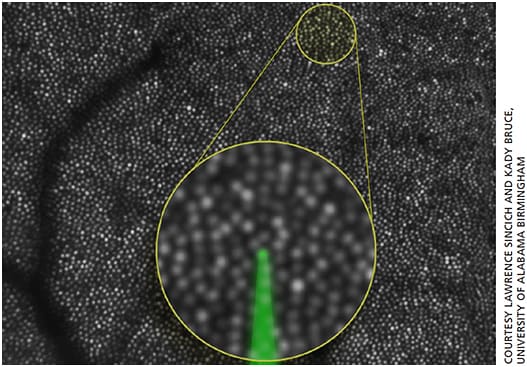
Figure 2. Human cone photoreceptor mosaic imaged in vivo with adaptive optics scanning laser ophthalmoscopy. Photoreceptors behave as optical waveguides, reflecting light within a narrow acceptance angle, allowing them to be imaged as brightly reflective spots. Real-time eye tracking helps to target and deliver light to features as small as photoreceptors, as illustrated by the green focused beam in the main figure and in the inset, which shows a magnified mosaic of cones 3.3 degrees from the foveal center. The dark branched structures are shadows of blood vessels.
Measuring functional activity is also the focus at the lab of Sheng-Kwei Song, PhD at Washington University in St. Louis, Mo. But here, the attention is on axons, and how they respond when injured. His lab’s tool of choice is diffusion MRI.
His team, Dr. Song says, thought it would be interesting to see if the damage to the axon can be quantified. The lab wants “to see what goes on with that location, and to measure the function of that location.”
“We are looking at axon integrity in pathology, which includes myelination,” says Dr. Song.
Reality check
Just because various diseases destroy photoreceptors doesn’t mean that finding a way to regenerate those photoreceptor cells will work for all diseases. “Replacing the cells in the retina may not be enough if [replacement] doesn’t fix the underlying problem,” says Dr. Steinmetz, using the example of chronic AMD — regeneration may bring back sight, but it may not last if the underlying disease process is not arrested.
“We don’t think we will come out with a single solution,” he says. So the NEI will continue to fund research to understand the basic biology of the retina, and that of diseases and their progression to different states. This way, researchers will “understand the similarity and differences among diseases so that appropriate therapies can be used.”
At the end of the day, researchers “will have a basic tool kit [to know] how to approach diseases, once they understand what causes [them],” says Dr. Steinmetz. “It’s important that we don’t focus entirely on efforts on just replacing cells, but [also] on an effort to understanding normal biology and the biology of these disease processes.”
Positive vibes
Dr. Dubra says that those involved feel highly motivated to attain this goal because advanced therapy treatments, like gene therapy for photoreceptor and neurodegenerative diseases, are already being tested in humans. Dr. Steinmetz agrees. “The reality of stem cells and gene therapy is really on the forefront in vision research,” he says, providing examples of Stargardt’s disease and AMD, whose trials involving embryonic stem cells to repair dead cells in the eye’s posterior have been successful.
Achieving routine non-invasive cellular imaging in patients would provide for more efficient testing of these drugs, Dr. Dubra says. “They could allow us to shorten the time required to see whether these treatments are working.”
Dr. Steinmetz, who left Johns Hopkins’ Zanvyl Krieger Mind/Brain Institute in 2003 to join the NIH, says the NEI’s audacious goal initiative, coupled with the Obama Administration’s BRAIN Initiative, makes this time in his career “thrilling.” “Many people believe that the retina will be the first circuit of the brain that is completely understood. We seem to be in the right place at the right time.”
Dr. Song, who is working with mice, sounded surprised when asked if the goal was attainable. “I think so, I sure hope so. It will be hard, [it will] not be easy.” OM
For the complete story, please visit ophthalmologymanagement.com
REFERENCES
1. Human eye. Encyclopaedia Britannica. http://www.britannica.com/science/human-eye.
2. NEI. Vision research needs, gaps, and opportunities https://nei.nih.gov/sites/default/files/nei-pdfs/VisionResearch2012.pdf.
3. Lin TH, Kim JH, Perez-Torres C, et al. Axonal transport rate decreased at the onset of optic neuritis in EAE mice. Neuroimage. 2014;100:244-253.