Focus on Diagnostics
How OCT improves measurement in cataract surgery
High-resolution axial imaging provides an advantage when evaluating corneal structures.
By Maolong Tang, PhD
The application of OCT in cataract surgery has many aspects, including preoperative screening of corneal1 and retinal diseases2, IOL power calculation3, intraoperative guidance of femtosecond laser cataract surgery4, as well as postoperative evaluation of IOL stability5 and other surgery-related ocular changes.6
Clinicians have quickly adopted OCT in a wide range of ophthalmic applications because its axial resolution is very high, ranging from 1 to 17 μm,7,8 making it ideal for imaging and measuring thin eye structures such as the retina and cornea.
This article will focus on the use of OCT for preoperative corneal power measurement and intraocular lens power calculation for cataract patients with previous laser vision correction (LVC) including LASIK and PRK.
LASER VISION CORRECTION
Traditional methods fall short
The curvatures of the anterior and posterior corneal surfaces determine the refractive power of the cornea. Traditionally, manual or automated keratometry or simulated keratometry from corneal topographers have been used to measure corneal power. By measuring the anterior corneal surface, these instruments can provide accurate corneal power measurements in normal eyes — that is, eyes that have not undergone LVC.
Actually, these methods do not directly measure the posterior corneal power; rather, they extrapolate it by assuming a keratometric index of 1.3375. The index is based on the assumption that anterior and posterior curvature has a fixed ratio of 0.883 (Gullstrand No. 1 schematic eye). It is lower than the corneal refractive index of 1.376 to compensate for the negative power of the posterior corneal surface.
This assumption of fixed ratio, however, is often wrong for corneas with pathologies or after LVC where changes in surface curvature mostly occur at the anterior corneal surface. The resulting errors in the measurement of corneal power by standard keratometry lead to difficulties in calculating IOL power after LVC. Poor cataract surgery outcome in post-LVC patients is a growing problem that is still waiting for a solution after years of active research because of this unique problem that LVC procedures introduce.
Clear delineation of boundaries
Direct measurement of posterior corneal power is a logical improvement to standard keratometry. The high axial resolution of OCT (Figure, page 41) allows clear delineation of both anterior and posterior corneal boundaries and direct measurement of posterior corneal power, which make it suitable for measuring corneal power.
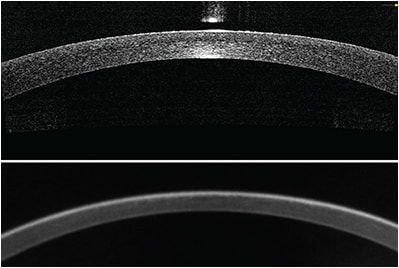
Figure: Central cornea imaged by a Fourier-domain OCT system (top). The image length is 6 mm and depth is about 2 mm. The eye had previous LASIK but the clinical history was not available. The corneal power was measured to be 38.18 D by automated keratometry. Bottom: The same eye imaged with a rotating Scheimpflug camera topographer. The image length and depth are also 6 mm and 2 mm, respectively. The top image (OCT) appears to have better depth and lateral resolution.
One report found OCT-measured anterior corneal power agreed well with standard keratometry,9 with repeatability of posterior corneal power measurement by Fourier-domain OCT of 0.02 D. OCT showed no significant change in posterior corneal power with LASIK, which suggested corneas remained stable after LASIK. However, the authors noted OCT-measured net corneal power was lower than standard keratometry. They attributed this variation to two factors:9
• The keratometric power was defined for the back vertex of the cornea, while net corneal power (or Gaussian equivalent power) was referenced to the principal plane of the cornea, which is slightly anterior to the front vertex.
• The assumed anterior/posterior curvature ratio of 0.883 by standard keratometry is higher than the actual ratio of 0.836 in normal eyes. The actual anterior/posterior curvature ratio for eyes after myopic LVC was even lower because of the decreased curvature on the anterior surface.
IOL POWER CALCULATION
Missing or incomplete data
Ophthalmic surgeons have proposed many methods of IOL power selection to avoid the inaccurate corneal power measurement by standard keratometry and improve refractive outcome after cataract surgery in post-LVC eyes. Most rely on available clinical tests that do not directly measure the posterior corneal power. They use the additional information, including the amount of LVC correction, LVC-induced change in manifest refraction change, or regression between standard keratometry and back-calculated corneal power to supplement standard IOL calculations.
These methods have been partially successful in reducing the unexpected refractive error after cataract surgery. However, because historical data from the time of LVC are often missing or incomplete, methods that rely on clinical history are not always applicable.
In the absence of a clearly superior method for measuring corneal power and calculating IOL power, a sensible approach is to use a wide variety of methods and derive a consensus value. ASCRS sponsored a website (www.iolcalc.org) that makes this ensemble approach easy, using a collection of formulae selected and organized by Drs. Warren Hill, Li Wang and Douglas Koch.
OCT forgoes historical data
Because Fourier-domain OCT provides direct posterior power measurements with high repeatability and does not require additional clinical history information, it has been used in IOL power calculation for post-LVC eyes.3,10 However, because standard IOL formulae were optimized toward standard keratometry and OCT-measured net corneal power were significantly lower than keratometry, it is not appropriate to use OCT-measured corneal directly in standard IOL formulae, so a different formula for OCT-based IOL calculation was developed.6 The OCT-based IOL formula is available at the author’s group website (www.coollab.net).
A pilot study of post-myopic LVC cataract surgery reported that the OCT-based IOL power calculation had better predictive accuracy than the Clinical History Method.10 In a larger study on post-LVC cataract surgery,3 OCT-based IOL calculation had a mean absolute error (MAE) in predicting refraction of 0.49 D in the post-myopic LVC group, compared to an MAE of 0.65 for the Haigis-L formula. Independent clinical studies, however, will be needed to confirm these results.
FUTURE OF OCT
Because OCT is a relatively new technology, we can anticipate many improvements in hardware and software in the near future to further refine corneal power measurement and IOL power selection. The reported studies used an OCT system with a speed of 20-40 kHz axial scan repetition rate and an axial resolution about 5 µm.
Future studies will likely use OCT systems with even higher performance. Scanning speeds up to 400 kHz axial scan repetition rate,11 and axial resolution of around 1 µm have been demonstrated in the laboratory. For the purpose of more precise corneal power measurement, improved speed and reduced motion error will be the main advantage for future OCT systems. This is a promising technology that could eventually become the best way to calculate IOL power for post-LVC cataract surgery. OM
REFERENCES
1. Nguyen P, Chopra V. Applications of optical coherence tomography in cataract surgery. Curr Opin Ophthalmol. 2013;24:47-52.
2. Creese K, Ong D, Zamir E. Should macular optical coherence tomography be part of routine preoperative cataract assessment? Clin Experiment Ophthalmol. 2012;40:e118-119.
3. Huang D, Tang M, Wang L, et al. Optical coherence tomography-based corneal power measurement and intraocular lens power calculation following laser vision correction (an American Ophthalmological Society thesis). Trans Am Ophthalmol Soc. 2013;111:34-45.
4. Bali SJ, Hodge C, Lawless M, et al. Early experience with the femtosecond laser for cataract surgery. Ophthalmology 2012;119:891-899.
5. Montes-Mico R, Cervino A, Ferrer-Blasco T. Intraocular lens centration and stability: efficacy of current technique and technology. Curr Opin Ophthalmol. 2009;20:33-36.
6. Kim SJ, Bressler NM. Optical coherence tomography and cataract surgery. Curr Opin Ophthalmol. 2009;20:46-51.
7. Drexler W, Fujimoto JG. Optical Coherence Tomography: Technology and Applications (Biological and Medical Physics, Biomedical Engineering). Berlin, New York: Springer, 2008.
8. Schuman JS, Puliafito CA, Fujimoto JG. Optical coherence tomography of ocular diseases, 3rd ed. Thorofare, NJ: SLACK Inc., 2012.
9. Tang M, Chen A, Li Y, Huang D. Corneal power measurement with Fourier-domain optical coherence tomography. J Cataract Refract Surg. 2010;36:2115-2122.
10. Tang L, Zeng GM, Shen GL, et al. Rapid detection of picloram in agricultural field samples using a disposable immunomembrane-based electrochemical sensor. Environ Sci Technol. 2008;42:1207-1212.
11. Potsaid B, Baumann B, Huang D, et al. Ultrahigh speed 1050nm swept source / Fourier domain OCT retinal and anterior segment imaging at 100,000 to 400,000 axial scans per second. Opt Express. 2010;18:20029-20048.
About the Author | |
![]() | Maolong Tang, PhD, is with the Casey Eye Institute, Oregon Health & Science University, Portland. His e-mail is tangma@ohsu.edu. |