How OCT helps monitor glaucoma progression
Improved evaluation of the key structures increases the sensitivity and specificity of detection.
By Theodore Lyu, MD, and Sung Chul Park, MD
To detect glaucoma progression, clinicians have widely used automated perimetry as a reference standard in the clinic and investigative trials.1-4 However, many eyes with glaucoma progression only show changes in the retinal nerve fiber layer (RNFL) or optic disc, or both, but not on visual field progression. This has been largely attributed to two factors: the intrinsic difference in the time course of structural and functional changes in glaucoma;5,6 and/or the difference in testing strategy between structural and functional tests.
In the Humphrey Field Analyzer (HFA; Carl Zeiss Meditec, Dublin, Calif.), one of the most popular perimetry machines, the visual field test points are spaced 6° apart,7 missing functional loss in the regions in between. However, current spectral-domain optical coherence tomography (SD-OCT) technology allows scanning of the entire optic disc and peripapillary area with a transverse resolution of approximately 15-20 µm (or 0.05-0.07°). Thus, sophisticated evaluation of the optic disc and RNFL structures will be valuable for increasing the sensitivity and specificity of glaucoma progression detection.
SD-OCT VS. TD-OCT
SD-OCT has advantages over the earlier iteration, time-domain OCT (TD-OCT), among them increased axial resolution (approximately 5 µm vs. 10 µm for TD-OCT), faster scanning speeds (around 25,000-55,000 A-scans/s vs. 100–400 for TD-OCT), reduced signal-to-noise ratio and increased reproducibility. These advantages of SD-OCT have enabled more reliable glaucoma progression analysis than TD-OCT.8,9 This article reviews the use of SD-OCT in detecting glaucoma progression, highlighting the various tools each SD-OCT machine offers.
IDENTIFYING PROGRESSIVE CHANGES
Event- and trend-based analysis
Evaluation of progressive changes of the RNFL or optic disc structures is based on two different principles:
• Event-based analysis identifies progression when the follow-up measurement exceeds a pre-established threshold for change from the baseline scan. The clinician can use this to identify a gradual change or an acute event that exceeds the threshold.
• Trend-based analysis calculates progression over time through a regression analysis of measurements, providing the ability to extrapolate the future rate of progression. The downside of the trend-based analysis is less deviation to acute changes.
Consistency of measurement
The chronic, degenerative nature of glaucoma requires consistency between the baseline and subsequent scans to accurately follow progression. Image registration minimizes misalignment between consecutive images, decreasing the variability in RNFL thickness measurements.
Guided Progression Analysis (GPA) was introduced in the third-generation TD-OCT (Stratus OCT, Carl Zeiss Meditec) in 2008. However, the Stratus OCT images analyzed in the GPA were not registered and thus it was impossible to perform reliable trend-based analysis. Moreover, difficulty in placing the scan circle in the same location weakened the performance of the Stratus OCT in detecting RNFL thickness change.
Different SD-OCT machines utilize different methods of image registration to maximize consistency between measurements. Spectralis OCT utilizes eye-tracking technology, which identifies retinal structures as reference points. The machine in subsequent scans recognizes these reference points and places the scan circle in the same location as the baseline scan.
Due to this eye tracking technology, Spectralis OCT can average up to 100 frames to generate one image, reducing the signal-to-noise ratio and artifacts substantially. The improved image quality enables more reliable and accurate RNFL thickness measurement and progression analysis. Spectralis OCT also incorporates the fovea-to-disc technology that orients the fundus anatomy and minimizes variability the patient's head orientation can cause.
Four SD-OCT devices
The four most commonly used commercially available spectral-domain OCT devices are:
• Spectralis OCT (Heidelberg Engineering, Carlsbad, Calif.).
• Cirrus HD-OCT (Carl Zeiss Meditec).
• RTVue-100 (Optovue, Fremont, Calif.).
• Topcon 3D-OCT 2000 (Topcon, Oakland, N.J.).
Each device has different glaucoma scan patterns and software for segmentation and progression analysis. What separates these machines are the set of benefits each brings, including degree of reproducibility, thickness and deviation maps for the RNFL and other retinal layers as well as automated trend- and event-based progression analyses.
The RTVue-100 registers blood vessels, which subsequent scans can use to place the scan circle at the same point. The Cirrus HD-OCT and Topcon 3D-OCT 2000 serially register pixels of the RNFL thickness maps over a 6 × 6 mm2 peripapillary area. On each visit, they automatically place the scan circle at the same location post-scan acquisition and compares pixels of subsequent scans against baseline scans to identify change.
EVALUATING RNFL THICKNESS
Circumpapillary RNFL thickness profile
Circumpapillary RNFL thickness measurement is the most commonly used imaging method for evaluating structural damage in glaucoma.
Spectralis OCT provides a change report with event-based progression analysis of circumpapillary RNFL thickness profiles and trend-based progression analysis of average and sector RNFL thickness measurements. This analysis graphically shows the location and amount of significant changes in the RNFL thickness profile from baseline as a red area. The trend-based analysis plots the serial global and six sectoral maps over time to assess the rate of change using linear regression analysis and shows differences from baseline.
Cirrus HD-OCT utilizes GPA to visualize RNFL changes and progression. It provides a visual display of the amount and location of significant change by comparing individual pixels of the follow-up images to the same pixels of the baseline image (event-based analysis). It also displays linear regression analysis of the average, superior and inferior RNFL thickness measurements (trend-based analysis).
RTVue-100 does not provide automated comparative analysis of circumpapillary RNFL thickness profiles, but it offers a figure with overlapped baseline and follow-up RNFL thickness profiles for easy comparison. The device also offers the Statistic Image Mapping module, which analyzes global RNFL thickness and six sectoral thicknesses. Based on these measurements, it performs a trend-based analysis to create a regression line to show a rate of progression.
Topcon 3D-OCT 2000 provides a linear regression analysis of average, superior and inferior RNFL thickness and graphical comparison of baseline and follow up RNFL thickness profiles. It also compares cup-disc ratio, cup volume and cup area between visits.
All four SD-OCT machines provide trend-based analysis of average and/or sector RNFL thickness. However, because of the localized nature of glaucoma progression, comparison of multiple RNFL thickness profiles obtained over time should be more sensitive in detecting subtle RNFL thickness changes (Figure 1, page 42).
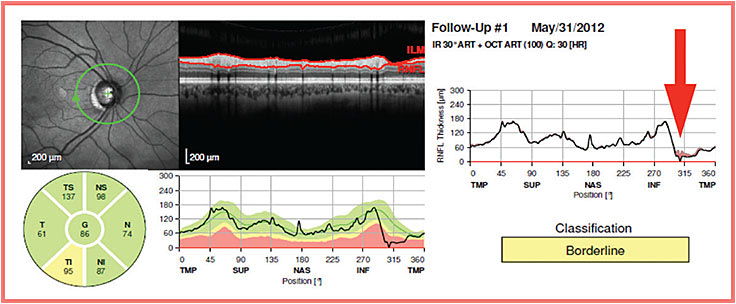
Figure 1. Because of the localized nature of glaucoma progression, longitudinal comparison of RNFL thickness profiles should be more sensitive in detecting glaucoma progression than global or sectoral RNFL thickness analysis.
RNFL thickness map
The RNFL thickness map is a reconstructed image of the distribution pattern of the peripapillary RNFL thickness. This map shows RNFL thickness along a color scale from thinner RNFL areas in blue to thicker RNFL in red. The circumpapillary RNFL thickness profile along the 3.46-mm scan circle cannot detect RNFL changes within or outside the scan circle.
Considering the most common locations of progressive RNFL thinning reside at the inferotemporal meridians approximately 2 mm, but not at 1.73 mm, from the optic disc center,10 the RNFL thickness map may have an additional diagnostic value to the circumpapillary RNFL thickness profile in detecting glaucoma progression.
Although other SD-OCT machines can display RNFL thickness maps, only the Cirrus HD-OCT can perform automated change analysis currently. Cirrus HD-OCT GPA computes the difference in RNFL thickness between the baseline scan (average of two baseline scans) and the follow-up scan (event-based analysis) and displays the area of change in the RNFL thickness change map. Cirrus HD-OCT also provides an RNFL deviation map by comparing the patient’s RNFL thickness map with a normative database (Figure 2). The RNFL deviation map help in detecting enlargement or deepening of RNFL defects by comparing serial deviation maps. It is more sensitive in detecting RNFL defects than the clock-hour and quadrant RNFL thickness maps.
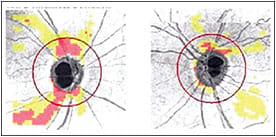
Figure 2. RNFL deviation map is helpful in detecting enlargement or deepening of RNFL defects inside and outside the circumpapillary RNFL scan circle.
EVALUATION OF THE MACULA
Macular thickness maps
Because glaucoma results in a decrease of retinal ganglion cells, which are most dense in the macula, evaluation of the macula can provide valuable information regarding glaucoma progression. While the Spectralis OCT measures the total macular thickness, the RTVue-100 segments the ganglion cell complex (RNFL, ganglion cell layer and inner plexiform layer) from the remaining retinal layers, and the Cirrus HD-OCT segments “ganglion cell layer + inner plexiform layer” from the remaining retinal layers.
Topcon 3D-OCT 2000 provides both a ganglion cell complex thickness map and a “ganglion cell + inner plexiform layers” thickness map. These machines display the thickness maps in a color-coded fashion, but none provide localized automated progression analysis of the macula in a localized fashion. Yet one can appreciate progressive thinning of the macular by comparing serial macular thickness maps.
Using the ganglion cell complex map of the RTVue-100, investigators in Hungary11 showed that ganglion cell complex parameters allowed for improved detection of early structural progression of glaucoma compared to RNFL thickness or optic nerve head parameters. Using the “ganglion cell + inner plexiform layers” analysis tool on the Cirrus HD-OCT, ophthalmologists in Korea12 examined glaucoma patients with severe visual field loss and demonstrated the rate of macular thinning was significantly faster in progressors than nonprogressors, but the rate of circumpapillary RNFL thinning did not differ significantly.
Interestingly, using the same strategy, the same group of investigators in Korea13 evaluated glaucoma patients with mild to moderate visual field loss and reported progressors exhibited significantly faster rates of macular and circumpapillary RNFL thinning than nonprogressors. These results suggested the potential utility of macular thickness map in detecting glaucoma progression and the limitation of circumpapillary RNFL thickness for detecting glaucoma progression in the later stages of the disease.
CONCLUSIONS
Using SD-OCT to assess peripapillary RNFL and macular layers can be valuable, in conjunction with visual field analysis, to improve our ability to detect glaucoma progression.14 Integrating results of peripapillary RNFL and macula analyses provides one way to improve the sensitivity and specificity to follow glaucoma progression.
Differences exist in imaging acquisition algorithms and analysis software among the various SD-OCT machines, and knowing these would be helpful in our ability to best utilize the devices clinically. Because of these differences, one should not use the measured values interchangeably among different SD-OCT devices to evaluate glaucoma progression.15
SD-OCT imaging of the lamina cribrosa holds a great promise to provide non-retinal ganglion cell structural parameters for detecting glaucoma progression, including focal lamina cribrosa defects16,17 and lamina cribrosa depth.18,19 OM
Limitations of SD-OCT
In progression analysis using SD-OCT, artifacts should be carefully detected and corrected. Except for the Spectralis OCT with its eye-tracking technology, motion artifacts can occur and interfere with accurate progression analysis.
Media opacity or irregular corneal surface can cause suboptimal OCT image quality that also affects the RNFL or macular thickness measurements. Coexisting posterior segment pathologies including epiretinal membrane and retinoschisis may cause overestimation of RNFL or macular thickness, leading to false-negative results. Additionally, clinicians should also consider age-related decline of RNFL and macular thickness when assessing glaucoma progression.
REFERENCES
1. Jampel HD, Singh K, Lin SC, et al. Assessment of visual function in glaucoma: a report by the American Academy of Ophthalmology. Ophthalmology 2011;118:986–1002.
2. Nouri-Mahdavi K, Nassiri N, Giangiacomo A, Caprioli J. Detection of visual field progression in glaucoma with standard achromatic perimetry: a review and practical implications. Graefes Arch Clin Exp Ophthalmol 2011;249:1593–1616.
3. Gordon MO, Beiser JA, Brandt JD, et al; Ocular Hypertension Treatment Study Group. The Ocular Hypertension Treatment Study: baseline factors that predict the onset of primary open-angle glaucoma. Arch Ophthalmol 2002;120:714–720.
4. Leske MC, Heijl A, Hyman L, et al; EMGT Group. Predictors of long-term progression in the Early Manifest Glaucoma Trial. Ophthalmology 2007;114:1965–1972.
5. Caprioli J. Correlation of visual function with optic nerve and nerve fiber layer structure in glaucoma. Surv Ophthalmol 1989;33(suppl):319-330.
6. Johnson CA, Cioffi GA, Liebmann JR, Sample PA, Zangwill LM, Weinreb RN. The relationship between structural and functional alterations in glaucoma: a review. Semin Ophthalmol 2000;15:221-233.
7. Heijl A, Patella VM. STATPAC. In: Haley MJ, ed. Essential Perimetry: The Field Analyzer Primer. 3rd ed. Dublin, CA: Carl Zeiss Meditec; 2002:40–70.
8. Wollstein G, Schuman JS, Price LL, et al. Optical coherence tomography longitudinal evaluation of retinal nerve fiber layer thickness in glaucoma. Arch Ophthalmol 2005;123:464–470.
9. Leung CK, Cheung CY, Weinreb RN, et al. Evaluation of retinal nerve fiber layer progression in glaucoma: a study on optical coherence tomography guided progression analysis. Invest Ophthalmol Vis Sci 2010;51:217–222.
10. Leung CK, Yu M, Weinreb RN, et al. Retinal nerve fiber layer imaging with spectral-domain optical coherence tomography: patterns of retinal nerve fiber layer progression. Ophthalmology 2012;119:1858–1866.
11. Naghizadeh F, Garas A, Vargha P, Hollo G. Detection of early glaucomatous progression with different parameters of the RTVue optical coherence tomograph. J Glaucoma 201;23:195-198.
12. Sung KR, Sun JH, Na JH, et al. Progression detection capability of macular thickness in advanced glaucomatous eyes. Ophthalmology 2012;119:308–313.
13. Na JH, Sung KR, Lee JR, et al. Detection of glaucomatous progression by spectral-domain optical coherence tomography. Ophthalmology 2013;120:1388–1395.
14. Medeiros FA, Leite MT, Zangwill LM, Weinreb RN. Combining structural and functional measurements to improve detection of glaucoma progression using Bayesian hierarchical models. Invest Ophthalmol Vis Sci 2011;52:5794–5803.
15. Pierro L, Gagliardi M, Iuliano L, et al. Retinal nerve fiber layer thickness reproducibility using seven different OCT instruments. Invest Ophthalmol Vis Sci 2012;53:5912–5920.
16. You JY, Park SC, Su D, Teng CC, Liebmann JM, Ritch R. Focal lamina cribrosa defects associated with glaucomatous rim thinning and acquired pits. JAMA Ophthalmol 2013;131:314-320.
17. Kiumehr S, Park SC, Syril D, Teng CC, Tello C, Liebmann JM, Ritch R. In vivo evaluation of focal lamina cribrosa defects in glaucoma. Arch Ophthalmol 2012;130:552-559.
18. Lee EJ, Kim TW, Weinreb RN, et al. Reversal of lamina cribrosa displacement after intraocular pressure reduction in open-angle glaucoma. Ophthalmology 2013;120:553–9.
19. Furlanetto RL, Park SC, Damle UJ, Sieminski SF, Kung Y, Siegal N, Liebmann JM, Ritch R. Posterior displacement of the lamina cribrosa in glaucoma: in vivo interindividual and intereye comparisons. Invest Ophthalmol Vis Sci 2013;54:4836-42.
About the Authors | |
![]() | Theodore Lyu, MD, is a clinical glaucoma fellow at New York Eye and Ear Infirmary of Mount Sinai, New York. His e-mail is tedlyu@yahoo.com. |
![]() | Sung Chul (Sean) Park, MD, is an assistant professor at the Icahn School of Medicine at Mount Sinai, and Peter Crowley Research Scientist, director of the Glaucoma Clinic and director of the Moise and Chella Safra Advanced Ocular Imaging Laboratory at New York Eye and Ear Infirmary of Mount Sinai. His e-mail is sungchulpark1225@gmail.com. |
Disclosures: Dr. Lyu reported no relevant conflicts. Dr. Park received instrument support and honoraria from Heidelberg Engineering, GmbH. |