Corneal Clarity
Journey Inside the Biofilm City
By Thomas John, MD
Bacteria have been able to sustain their existence for millennia through many ingenious defense mechanisms. This month, we delve into the formation of biofilms and the ways in which they shield bacteria from their adversaries. You can imagine a biofilm as a complex, well-organized city of microorganisms that are sheltered from adverse influences like antibiotics and human immune cells, rendering them ineffective. Bioflim is bacteria's ultimate shelter!
My interest in biofilm dates back to my clinical cornea fellowship days at Harvard Medical School, Massachusetts Eye and Ear Infirmary in Boston, where — under the guidance of Dr. Kenneth R. Kenyon — we studied bacterial adherence and biofilm with relationship to contact lenses. In fact, contact lens biofilms may play a role in contact lens-related bacterial keratitis and corneal ulcerations, as I'll explain (Figure 1). And that's just the start of biofilm's relationship to ophthalmology.
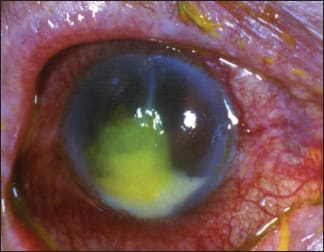
1. Bacterial corneal ulcer with a hypopyon. COURTESY OF THE AUTHOR.
Before we delve into that, though, I'll start with the basics of biofilm. I shall explain biofilm as it relates to the eye, the human body and the environment that we live in.
Examining the What and Why
Earth has been estimated to be about 4.6 billion years old, and man has existed for about 100,000 to 200,000 years; at what point biofilms came into existence is hard to pinpoint. Suffice it to say that biofilms have possibly been around for several centuries, but it was not discovered until recently, by microbiologist William Costerton in 1978.
Biofilms protect bacteria from antimicrobial treatments, thus causing a significant health hazard. So while microbiologists have focused on free-living bacteria for the past 300 years, it is time now to turn our attention to stationary or planktonic bacteria; namely, biofilm or slime (not all slime are biofilm).
Biofilms occur when free-floating bacteria attach to a surface. These bacteria secrete extracellular polymeric substances (EPS), also known as exopolysaccharides, that form a structural matrix, which helps in bacterial adherence and encases these bacteria. A fully mature biofilm is multi-layered, with a matrix of EPS that often has vertical towers or mushroom-like structures of microorganisms, separated by interstitial spaces, and a conditioning film. The interstitial spaces provide a two-way channel where nutrients are absorbed into the bio-film from the surrounding liquid and byproducts are moved out of the biofilm.
Thus, biofilm is a collection of microorganisms where cells adhere to each other on a surface that is in contact with water, forming colonies of bacteria and other microorganisms such as fungi, yeasts and protozoa. Biofilms can form on both solid and liquid surfaces, and on soft tissues (e.g., diabetic ulcer).
Once within the biofilm domain, we are looking at stationary bacteria or planktonic bacteria. Biofilms can be found in domains from medical to industrial to the very homes we live in. Hence, we need to shift our attention from free-floating bacteria to planktonic bacteria. The EPS not only holds the biofilm together, but also protects the bacteria within the biofilm and, via biochemical signals, allows communication among bacteria. Biofilms may also contain water channels that allow nutrient distribution and signaling molecules. It is interesting to note that bacteria of the same species differ when they are free-floating as compared to being stationary within a biofilm.
As such, a biofilm is a functional collection of microorganisms well organized within an elaborate, exopolymer matrix. Formation of a biofilm may be thought of as a change in bacterial lifestyle from living as individual organisms to a group or community living structure.
How Does Biofilm Form and Propagate?
Let's walk through the biofilm life cycle. Biofilm formation is a complex process, but may be somewhat simplified by compartmentalizing it into three major stages:
1. Formation
2. Development
3. Dispersion
In formation, the initial triggering factor is the transformation of a surface not conducive to bacterial attachment into a surface that permits and promotes bacterial adherence. In other words, the conditioning films alter the substratum surface properties to allow microbial adherence to the changed surface. This initial adherence is via weak, reversible adherence through Van der Waals forces of attraction and repulsion. These initial colonies provide more diverse adhesion sites and help build the matrix that holds the biofilm together.
With this commencement of colonization, a biofilm grows via cell division and recruitment. Thus, free-floating bacteria become stationary, or planktonic, bacteria that build their own city, protected from all noxious and adverse elements. Hence, the first step is bacterial attachment to a surface. This is followed by cell-to-cell adhesion and bacterial pluristratification onto the substratum. Biofilm formation is partly controlled by quorum sensing, an interbacterial communication mechanism that is dependent on population density. Within this biofilm, efficient horizontal transfer of resistance and virulence genes occur.
During development, the final stage of biofilm formation, the biofilm is well established. The only further alterations can be a change in shape and size of the biofilm.
Finally, dispersion is a mechanism by which biofilm propagates. It helps biofilm to spread and colonize.
Biofilm and the Environment
In nature, biofilms are often found on solid substrates that are immersed or exposed to an aqueous solution. Additionally, they can form as free-floating mats on liquid surfaces; in high humidity climates, they can form on the surface of leaves. This type of existence makes us feel that biofilms are ubiquitous in nature. They are present on pebbles and rocks beneath most rivers and streams, on the surface of stagnant pools, inside water and sewage pipes, pipelines of offshore oil and gas, they are the slimy coating that fouls tanks and boat hulls.
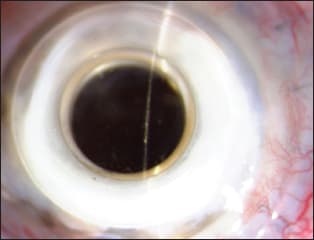
Figure 2. Bacterial biofilm in an artificial cornea (keratoprosthesis). COURTESY OF THE AUTHOR.
Biofilms can form and grow in extreme environments, from hot springs to frozen glaciers. In the home environment, biofilm can grow in showers since they provide a warm and moist environment that facilitates formation. In food preparation areas, a biofilm may form on floors and counters, and can make sanitation difficult. They can also form in cooling or heating-water systems, hampering effective heat transfer.
Although biofilms in general are not welcome, they do have beneficial applications, such as treating sewage, contaminated soil and industrial waste. In sewage treatment, wastewater may be allowed to pass over biofilms grown on filters that extract and digest organic compounds. Also, biofilms can assist in eliminating petroleum oil that may contaminate oceans or marine systems. Biofilms on slow sand filters help filter water from rivers, lakes and springs.
Biofilm and Medicine
Biofilms are present on teeth as dental plaques that can lead to decay and gum disease. Patients with cystic fibrosis often get Pseudomonas infection that may lead to the formation of biofilm and secondary bacterial resistance to antibiotics. According to the Centers for Disease Control estimates, more than 65% of hospital-acquired (nosocomial) infections are due to biofilms.
Biofilms play a role in infections, including urinary tract and catheter-related infections, infections associated with central venous catheters, gingivitis, middle-ear infections, endocarditis, infections in patients with cystic fibrosis, chronic sinusitis and gonococcal cervical infections. Biofilms can also cause infections associated with implanted devices such as heart valves, coronary stents, pacemakers, neurosurgical ventricular shunts, implantable neurosurgical stimulators, intrauterine devices, inflatable penile implants, breast implants, cochlear implants, intraocular lenses (see below), dental implants, arthro-prostheses and fracture-fixation devices.
In addition to bacterial biofilms, fungal biofilms can also contaminate implanted medical devices. They can result in life-threatening systemic infections, especially in immunocompromised individuals. In the medical field, about one million cases of catheter-associated urinary tract infections are reported yearly, many of which are thought to be due to biofilm-associated bacteria.
Biofilms can also have a deleterious effect on cutaneous wounds — that is, they can retard wound healing and decrease the efficiency of topical antibacterial agents in treating infected cutaneous wounds. Fungal biofilms can cause chronic vaginal infections.
Its Role in Ophthalmology
In the ocular arena, bacterial biofilms have been demonstrated in contact lenses, intraocular lens implants, scleral buckles and suture materials. Biofilms may be present in both the contact lens and contact lens cases. Similarly, the abiotic substrate of a punctal plug may be the site of biofilm formation. Conjunctivitis has been associated with punctal plugs with bacterial and fungal biofilm. Other prosthetic devices such as the artificial cornea may be secondarily colonized by bacteria and result in biofilm formation (Figure 2). Lamellar procedures such as posterior lamellar keratoplasty can be a route of potential bacterial introduction and deep stromal infection with biofilm formation (Figure 3).
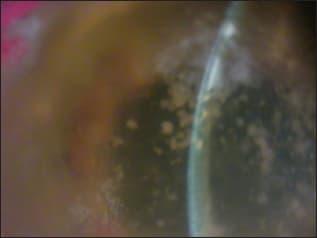
Figure 3. Intrastromal bacterial biofilm following deep lamellar endothelial keratoplasty (DLEK). COURTESY OF THE AUTHOR.
Also, biofilm formation has been associated with delayed postoperative endophthalmitis and in crystalline keratopathy. Infectious crystalline keratopathy (ICK) is a chronic, corneal infection with branching, white crystalline, corneal opacities; it is usually accompanied by minimal ocular inflammation. The most common cause of ICK is Streptococcus viridans. ICK can be associated with biofilm formation. Candida albicans has been associated with biofilm formation in ICK.
Sight-threatening endophthalmitis is usually caused by gram-positive organisms such as Staphylococcus epidermidis. It is often the result of postoperative and post-traumatic bacterial access to ocular posterior segment and can lead to significant, permanent visual compromise or complete loss of vision. However, an alternative infective modality is endophthalmitis caused by bacterial biofilms that form on the surface of intraocular lens implants. More work needs to done in this area of Staphylococcus aureus and Staphylococcus epidermidis len-sassociated biofilms that lead to endophthalmitis.
Additionally, because biofilm shelters bacteria, when present within the eye they make it more difficult for ocular antibiotics to succeed in preserving vision. Bacteria within the shelter of biofilm is thought to be up to 1,000 times more resistant than the same bacteria that is not sheltered by the biofilm.1-3
When such a biofilm forms within the body and contaminates prosthetic implants, antibiotics are ineffective, and often the implant may have to be removed. Hence, biofilm's impact in the human body is quite significant.
Future Directions
Because antibiotics — man's only major weapon against infective microorganisms — are ineffective against microorganisms living within the microbial city of a biofilm, we need substances that can fight biofilm — armaments that can destroy the microbial city itself. Although research efforts continue, not many effective anti-biofilm substances are currently available.
Some paths currently under consideration:
Biofilm matrix-degrading enzymes have been shown to inhibit growth of biofilm colonies and induce dispersion, and macrolide antibiotics such as azithromycin have been shown to impair biofilm production. Developing prosthetic devices that are resistant to bacterial biofilm formation may help in the continued war against microorganisms. Additionally, treatments that inhibit transcription of biofilm-controlling genes may help in reducing biofilm-related infections. There is some evidence to suggest lactoferrin that sequesters iron can prevent biofilm formation on the ocular mucosal surface. Iron is an essential nutrient for biofilms, and a shortage of iron means the bacteria go elsewhere to establish their elaborate biofilm. Also, nitrous oxide has been shown to kill bacteria and fungi growing planktonically in a biofilm.
Hopefully, continued research efforts will show us new and effective ways to penetrate biofilm, kill biofilm-bacteria and shatter the bacterial shelter to improve the environment, advance the industrial field, preserve vision and promote health for man's survival against microorganisms in the 21st century. OM
References
1. Prosser BL, et al. Method of evaluating effects of antibiotics on bacterial biofilm. Antimicrob. Agents Chemother. 1987 Oct; 31(10):1502-1506.
2. Gristina, AG, Hobgood CD, Webb LX, Myrvik QNl. Adhesive colonization of biomaterials and antibiotic resistance. Biomaterials. 1987 Nov;8(6):423-426.
3. Thien-Fah C. Mah and O'Toole GA. Mechanisms of biofilm resistance to antimicrobial agents. Trends Microbiology. 2001 Jan;9(1):34-39.
![]() |
Thomas John, MD, a world leader in lamellar corneal surgery, is a clinical associate professor at Loyola University at Chicago, and in private practice in Oak Brook, Tinley Park and Oak Lawn, III. E-mail him at tjcornea@gmail.com. |