Artificial Vision Implants: Will Science Fiction Become Scientific Fact?
Early breakthroughs bode well for this promising technology.
By Rodrigo A. Brant Fernandes, MD, and Mark S. Humayun, MD, PhD
After decades of research from different groups, and clinical trials from several centers around the world, the first implantable retinal prosthesis became commercially available in Europe this February. The Argus II from Second Sight (www.2-sight.com) is designed to restore some visual perception to patients with severe outer retinal degenerations like retinitis pigmentosa and AMD.
Currently, the best vision obtained with the 60-electrode/channel Argus II implant is 20/1262.1 Although this technology has not yet reached the level of artificial vision popularized in science fiction, it has the potential to be transformative in the lives of well-selected patients. Future developments are necessary to improve vision to the point where it can restore reading or face-recognition vision. The development of a higher resolution implant is dependent on both engineering breakthroughs and greater understanding of the pathophysiology of the diseased retina. Herein, we provide a brief overview of some of the more critical issues.
Remodeling in Degenerate Retina
To understand how a retinal prosthesis can stimulate the diseased and remodeled retina, it is important to understand the stages of retinal degeneration and the associated anatomical and physiological changes that occur. Post-mortem morphometric study of the retinas of patients with endstage RP has revealed that 78.4% of inner nuclear and 29.7% of ganglion layer cells were retained, compared to only 4.9% of photoreceptors.2-4 Also, 93% of retinal ganglion cells were spared and an increase in inner nuclear layer cells (by 10%) was noted in legally blind neovascular AMD patients.5-7 However, a subsequent study by Marc et al. pointed out that, although there might be remaining retinal neurons in these advanced photoreceptor conditions, the retina is remodeled.
Figure 1. Location of the array can be either epiretinal or subretinal.
The authors described three phases of such remodeling.8 In the first two, photoreceptor stress, death and associated loss of tropic transport were observed. Muller cells can form a dense fibrotic layer and seal off the subretinal space, which could result in a barrier for electrical stimulation from implants placed subretinally. In phase 3, the number of viable cells of all classes is depleted. Bipolar and amacrine cells can migrate up to the ganglion cell layer and undergo neural rewiring.
Despite such histological findings, the work of Humayun et al. has demonstrated that even when there is profound photoreceptor loss in advanced RP and AMD, controlled electrical stimulation applied epiretinally (on the ganglion side of the retina) results in localized predictable visual sensations in blind patients.9-10 These short-term results in humans led to the continued enthusiasm of many to continue to develop retinal prostheses.
Technology
The devices in this class are considered visual prostheses — implants that are able to elicit visual perceptions, or “percepts,” in a patient through electrical stimulation of the visual system at a particular location, such as the visual cortex, visual pathway, optic nerve or neural retina. Such electrically-elicited percepts have been termed phosphenes.
The most successful neural prosthesis to date has been the cochlear implant — an implant already in commercial use, and a model for the development of the retinal prosthesis being studied today.11
Similar in many ways to the placement of the cochlear implants, the flexible retinal electrode array can be placed on the retinal surface, on the subretinal space and even in the suprachoroidal space (Figure 1). The device is comprised of an electrode array, a cable and an external processing device. The photons can be captured either by a camera mounted in glasses or inside the eye or with the use of an implanted microphotodiode.
Epiretinal Prosthesis
The epiretinal implants already in clinical trials consist of an intraocular electrode array in contact with the nerve fiber layer, connected by a cable or wirelessly to a microprocessor and power source, which are connected to an image device, such as a camera, to capture the image.
The camera captures the image and sends it to the micro processor, which processes the data and sends the impulses to stimulate the retina to the electrode array placed on the surface of the retina. Future designs will have more components placed intraocularly (i.e., electronics and camera), but in the Argus II system, many of these remain outside the eye (Figure 2).
The concept and implantation is the same as in other epiretinal devices studied in the past, and is the only FDA-approved study. The Argus devices have the longest follow-up data, with six patients implanted in the first trial version (Argus I, 16 electrodes), and 32 in the Argus II trial. Also, it is the only retinal implant to receive a CE Mark in Europe, and the first commercially available implant in the world.
Figure 2. Schematic of epiretinal prosthesis with external camera. Data and power are wirelessly transmitted via an inductive link. A flexible electrode array is placed on the retinal surface.
Patients using the Argus II system wear a pair of glasses on which a miniature camera has been mounted, plus a video processing unit that can be clipped to a belt. The external components aquire and process images, then transmit the signal to a 60-channel stimulating microelectrode array implanted epiretinally (Figure 3). The implantation procedure is akin to a pars plana vitrectomy plus 360° scleral buckle, with the cable and electrode inserted via pars plana.
A prospective, single-arm, nonrandomized trial with three years of follow-up per subject (www.clinicaltrials.gov, NCT00407602), enrolled 32 patients with severe-to-profound outer retinal degeneration (e.g., RP, choroideremia). Subjects were recruited from 11 centers in five countries. Average patient age at implantation was 58 ±10 years (range, 27-77). The research is ongoing; at present the duration of the implant has been 18 ± 10 months (range, 7-33 months), and the cumulative duration is more than 60 subject-years.1
Visual acuity of subjects was measured using high-contrast four alternative choice square-wave grating tests for both Argus I and II subjects. The best performing Argus I subject had a visual acuity of 20/4000.12 Patients' best visual acuity with the Argus II was 20/1260.1
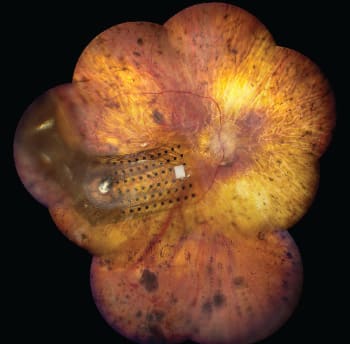
Figure 3. The Argus II 60-channel electrode array on the surface of the retina.
A second epiretinal device unaffiliated with the Argus series is the Epiret3 (www.epiret.de). Its developers studied a 25-electrode device, and recently presented data on an acute clinical trial.13 Six legally blind patients with RP were included in the study, and all subjects reported visual percepts when stimulated by the implant. Activation of the implant to record visual sensations was performed during three sessions around postoperative days 7, 14 and 27, respectively, and at day 28 the implant was removed.13
A third implant is the 49-electrode epiretinal device from Intelligent Medical Implants (www.imidevices.com). This device is currently under clinical investigation in a multicenter trial. The main findings of the chronic implant clinical trial are low thresholds to elicit visual perceptions, including form vision, and that the implant is reasonably well tolerated by the eye. The device was only activated in the clinic and directly controlled by computer (i.e., no camera). Most implants were removed after a few months, but some have stayed in place for several years.14
Subretinal Prosthesis
The Artificial Silicon Retina (ASR) is a subretinal implant designed to replace the degenerated outer retinal layers by capturing the light stimuli and electrically stimulating the ganglion and bipolar cell layers. It involves the implantation of a subretinal microphotodiode array with stimulation electrodes adjacent to each microphotodiode, making it possible to capture the light and subsequently stimulate the retina in the same region of the received photons. The implantation can be done intraocularly, through a pars plana vitrectomy and retinotomy (ab interno), or via a transscleral approach (ab externo). In the ab externo case, because of the large choroidal incision and risk of subretinal bleeding, the procedure is performed under hypotensive anesthesia.
The ab interno subretinal approach was initially championed by Chow et al.15-17 Their claim was that a simple photodiode (solar cell), without external electronics, power or external camera, would be sufficient. Work performed by Zrenner et al.18 has largely proved this simplistic approach to be not feasible as it lacks both power and data regulation. In fact, Chow et al. themselves now have abandoned the notion that their approach is a prosthetic but are now claiming that the low level of current delivered from the implant, although insufficient to electrically activate the remaining retinal neurons in a photoreceptorless retina, may be enough to be therapeutic and neuroprotective to otherwise dying retinal photoreceptors.
Optobionics implanted the ASR device in 10 patients in a single-center study, followed by 20 subjects in a multi-center study. A detailed report on the first six subjects was published in 2004,19 with follow-up reports via conference abstracts. The implants are 3 mm in diameter with 3500 electrodes and deliver current to the retina when light is incident on the implant. The authors concluded that the subretinal ASR implant was not directly mediating artificial vision (i.e., electrical stimulation of retinal neurons triggering visual perception); rather, its presence in the subretinal space was through an “indirect effect,” possibly through release of growth factors, improving the health of the retina.20 Unfortunately, Optobionics' trial did not meet its endpoint and the company had to sell off most of its assets.
Zrenner et al. at Tübingen University have continued to develop a subretinal prosthesis called the Retina Implant AG, which consists of a microphotodiode array (Figure 4) powered by either a laser or telemetry for the replacement of degenerated photoreceptors in outer retinopathies.18,21,22
In a prospective, open-label, placebo-controlled trial (www.clinicaltrials.gov, NCT00515814) that lasted approximately four months, 14 subjects received the implant.23 For the placebo arm, the system was switched off. Response to visual stimumi was tested soon after implantation. The study authors reported data for only three of the original 14 patients, who had lost vision to either RP or choroideremia. The maximum visual acuity of the best subject was 20/1000 (logMAR = 1.69).21
Looking Into the Future
Although the early studies differed somewhat in their outcomes, one consistent finding is that increasing the number of electrodes and processing capacity im proved the visual acuity of the im planted patients. But is there a limit to that? So far, the increase in the number of pixels corresponded to an in crease in the visual acuity. When the first Argus I implant was placed (16 electrodes), the research community thought that visual acuity achieved with the system was already maximal, but with the Argus II (60 electrodes) an improvement was reported.
Figure 4. The Retina Implant AG in place. FROM ZRENNER E, ET AL. SUBRETINAL ELECTRONIC CHIPS ALLOW BLIND PATIENTS TO READ LETTERS AND COMBINE THEM TO WORDS. PROC. R. SOC. B. BIOL. SCI. PUBLISHED ONLINE 3 NOVEMBER 2010. REPRINTED WITH PERMISSION OF ROYAL SOCIETY PUBLISHING.
However, in the Retina Implant AG trials, the 1500-pixel device translated into only a small gain in visual acuity. Could it be that the histological changes described earlier in this article affected the subretinal Retina Implant AG device more deleteriously than the epiretinal Argus II implant?
The Second Sight team is already planning an upgraded device with more condensed electrodes and higher visual processing capability, with a goal of reaching 1000 electrodes in the future. If the trend of increasing the electrode volume leading to greater acuity gain proves accurate, it may be possible to achieve reading ability and facial recognition.
Researchers and clinicians involved in these efforts no doubt have much work ahead of them, as replicating the function of the human eye is among the most complex and ambitious tasks our profession and related disciplines have undertaken. However, the payoff from developing such technology is also undoubtedly tremendous from both an economic and humanitarian standpoint. To realize the full potential of these technologies, we have to allow time not only for clinical and biological testing but also continued engineering and technical advances. Nevertheless, the future looks bright for this technology — and hopefully for the patients it may serve one day. OM
References
1. Humayun MS, et al. Interim performance results from the Second Sight Argus TM II Retinal Prosthesis Study. Paper presented at: The 2011 ARVO Annual Meeting; May 3, 2011; Fort Lauderdale, FL. Abstract 2594.
2. Humayun, MS, et al. Morphometric analysis of the extramacular retina from postmortem eyes with retinitis pigmentosa. Investigative Ophthalmology & Visual Science. 1999;40(1):143-8.
3. Santos, A, et al. Preservation of the inner retina in retinitis pigmentosa. A morphometric analysis. Archives of Ophthalmology. 1997;115(4):511-5.
4. Stone, JL, et al. Morphometric analysis of macular photoreceptors and ganglion cells in retinas with retinitis pigmentosa. Archives of Ophthalmology. 1992;110(11):1634-9.
5. Curcio, C.A., et al. Photoreceptor loss in age-related macular degeneration. Investigative Ophthalmology & Visual Science. 1996;37(7):1236-49.
6. Kim, SY, et al. Morphometric analysis of the macula in eyes with geographic atrophy due to age-related macular degeneration. Retina. 2002;22(4):464-70.
7. Kim, S.Y., et al., Morphometric analysis of the macula in eyes with disciform age-related macular degeneration. Retina. 2002;22(4):471-7.
8. Marc RE. Neural remodeling in retinal degeneration. Prog Retin Eye Res. 2003;22:607.
9. Humayun, MS, et al. Visual perception elicited by electrical stimulation of retina in blind humans. Archives of Ophthalmology. 1996;114(1):40-6.
10. Humayun, MS, et al. Pattern electrical stimulation of the human retina. Vision Research. 1999;39(15):2569-76.
11. Wilson BS, et al. Cochlear implants: current designs and future possibilities. J Rehabil Res Dev. 2008; 45(5):695-730.
12. Caspi A, et al. Feasibility study of a retinal prosthesis:spatial vision with a 16-electrode implant. Arch Ophthalmol. 2009;127(4):398-401.
13. Klauke S, et al. Stimulation with a Wireless Intraocular Epiretinal Implant Elicits Visual Percepts in Blind Humans. IOVS. 2011;52(1).
14. Richard G. Long-Term Stability of Stimulation Thresholds Obtained From a Human Patient With a Prototype of an Epiretinal Retina Prosthesis. Invest Ophthalmol Vis Sci. 2009; E-Abstract 4580.
15. Chow AY, et al. Safety, feasibility and efficacy of subretinal artificial silicon retina prosthesis for the treatment of patients with retinitis pigmentosa [abstract]. Invest Ophthalmol Vis Sci. 2002; E-abstract 2849.
16. Chow AY, et al. Subretinal implantation of semiconductor-based photodiodes: durability of novel implant designs. J Rehabil Res Dev. 2002;39(3):313-21.
17. Chow AY, et al. The artificial silicon retina microchip for the treatment of vision loss from retinitis pigmentosa. Arch Ophthalmol. 2004;122(4):460-9.
18. Zrenner E, et al. The development of subretinal microphotodiodes for replacement of degenerated photoreceptors. Ophthalmic Res. 1997;29:269-280.
19. Chow AY, et al. The artificial silicon retina microchip for the treatment of vision loss from retinitis pigmentosa. Arch Ophthalmol. 2004;122(4):460-9.
20. Ciavatta VT, et al. Retinal expression of Fgf2 in RCS rats with subretinal microphotodiode array. Invest Ophthalmol Vis Sci. 2009;50(10):4523-30. Epub 2009 Mar 5.
21. Zrenner, E. Et al. Subretinal electronic chips allow blind patients to read letters and combine them to words. Proc. R. Soc. B. Published online 3 November 2010.
22. Besch D, et al. Extraocular surgery for implantation of an active subretinal visual prosthesis with external connections: feasibility and outcome in seven patients. Br J Ophthalmol. 2008;92:1361-1368. doi:10.1136/bjo.2007.131961.
23. Zrenner E, et al. Details on the technology of the subretinal implant, clinical study design, results and spontaneous reports of patients including nine movie clips on performance. Electronic supplementary material to Proc Biol Sci. 2011;22; 278(1711):1489.
![]() |
Rodrigo A. Brant Fernandes, MD, is from the Doheny Eye Institute, Keck School of Medicine at the University of Southern California in Los Angeles and the Federal University of Sao Paulo, UNIFESP-EPM, in Sao Paulo, Brazil. He has no financial interest in the products or companies mentioned herein. Dr. Brant may be reached at rbrant@doheny.org. |
![]() |
Mark S. Humayun, MD, PhD, is from the Doheny Eye Institute, Keck School of Medicine, University of Southern California in Los Angeles. He has equity in, is a patent holder for, receives royalties from, and is a consultant to Second Sight Medical Products, Inc. Dr. Humayun may be reached at humayun@usc.edu. |