How Corneal Biomechanics Affect IOP Measurements
Measuring these biomechanical parameters provides intriguing diagnostic clues.
BY LEON W. HERNDON, MD
Elevated intraocular pressure is the major modifiable risk factor for the development1 and progression2 of glaucoma. The Goldmann applanation tonometer is currently the gold standard for measuring IOP. In first describing their applanation tonometer, Goldmann and Schmidt discussed the effect of central corneal thickness (CCT) on IOP as measured by the new device.3 They felt that variations in corneal thickness occurred rarely in the absence of corneal disease and assumed a CCT of 520 μm but acknowledged that, at least theoretically, CCT might influence applanation readings.
This article will discuss efforts to examine the role of corneal biomechanics in influencing applanation IOP measurements and the prediction of disease progression.
Goldmann and Schmidt started from the hypothesis that the cornea might be considered as a sheath covered by two membranes between which almost non-shifting water is located. It has since become apparent that CCT is more variable among clinically normal patients than Goldmann and Schmidt ever realized. Von Bahr4,5 showed that there were large variations in CCT within a normal population and Ehlers and co-workers2 demonstrated that this variation in CCT had an effect on applanation-measured IOP. Many studies have since looked at the influence of CCT on IOP measurement, with most agreeing there is an increase in measured IOP with increasing CCT.6 However, CCT alone accounts for only a small proportion of the inter-individual variation in measured IOP.
The Role of the ORA
Goldmann applanation tonometry measures IOP by flattening the cornea, which is not neutral in this measurement. Liu and Roberts7 have shown that factors affecting corneal resistance include structural considerations such as the amount of rigidity produced by the way the collagen beams in the tissue line up.
The “bendability” of corneal tissue can also be affected by short-term factors such the presence of corneal edema. Reichert's Ocular Response Analyzer (ORA) (Figure 1) measures the corneal response to indentation by a rapid air pulse. (Figure 2) The principles of the ORA are based on those of non-contact tonometry, in which the IOP is determined by the air pressure required to applanate the central cornea.
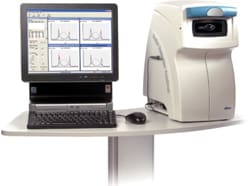
Figure 1. The ORA is used to make biomechanical measurements of the cornea.
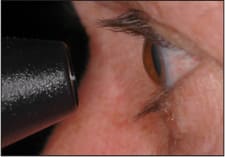
Figure 2. The air-pulse test to determine corneal hysteresis is very simple.
The ORA makes two measurements of the corneal response to the pulse of air — the force required to flatten the cornea as the air pressure rises (force-in applanation, P1) and the force at which the cornea becomes flat again as the air pressure falls (force-out applanation, P2). The difference between the two is termed corneal hysteresis (CH).
Corneal Hysteresis and Corneal Resistance
A feature of the ORA is that the maximum air pressure applied is not constant and is dependent on P1, a value determined by both the true IOP and the structural resistance of each individual eye. Kotecha et al9 recently assessed what effect this feature might have on CH values by measuring eyes before and after pharmacological reduction of IOP. The study found a weak, but significant, negative correlation between changes in CH and changes in IOP, such that higher values of CH were found at lower levels of IOP. Variations in maximum air pressure applied to the cornea may cause differing amounts of corneal indentation. Further work is needed to determine whether these variations alter the CH measurement.
The same study also assessed the relationship between changes in P1 and P2 following the medical lowering of IOP and found that for every unit change in P1 there was a corresponding, proportional change in P2. This relationship was used to determine k for the equation P1-kP2 to derive a parameter termed the corneal constant factor (CCF). Both the CCF and CRF are corneal parameters that are relatively unaffected by IOP, unlike CH. In this study, CH, CCF and CRF were positively associated with CCT, suggesting that thicker corneas exhibit greater viscoelastic properties. There was a negative association between both CCF and CRF and advancing age, possibly due to an age-related increase in cross-linking of collagen fibrils within the cornea leading to a stiffer structure.
Some Diseases/Procedures Reduce CH
Corneal hysteresis has been shown to be reduced in patients with keratoconus and Fuchs' endothelial dystrophy, conditions that cause a progressive decrease and increase in CCT, respectively. This finding may be an indication of the bio-mechanical similarities of such corneas in which alterations of stromal lamellar organization are known to occur.10,11 CH has also been shown to be markedly reduced in children with congenital glaucoma, especially in those with marked Haab's striae and larger corneal diameters.12 Pepose et al.13 have shown that CH and CRF show a marked reduction following LASIK. This change in corneal biomechanical properties may be a result of the creation of the LASIK flap altering anterior stromal lamellar organization.
Implications for Future Research
Researchers have investigated the possibility that a thick or thin cornea may tell us something about the back of the eye. It's been noted, for instance, that African-Americans have thinner corneas than Caucasians and often have more advanced glaucoma,21-23 so it's possible that a thinner cornea may indicate a thinner or more susceptible lamina cribrosa or optic nerve. Other research has found that ocular hypertensive patients with thinner corneas have thinner nerve fiber layers than ocular hypertensive patients with thicker corneas and healthy control subjects.24
It is also possible that differences in corneal biomechanics may indicate more generalized structural differences between eyes. Wells et al.25 investigated the relationship between acute IOP-induced optic nerve head deformation and CH and CCT in normal and glaucomatous eyes. They found that in glaucoma patients CH, but not CCT, was associated with increased deformation of the optic nerve surface during transient elevations of IOP.
This finding did not hold true in control patients, suggesting that glaucoma may modify the biomechanical properties of tissues supporting the optic nerve head. Quigley and colleagues26 and Hernandez27 have reported that there are alterations in the elastin of the optic nerve head in both human and experimental glaucoma and have suggested that differences in elastin function may have a part to play in susceptibility to glaucomatous injury. Congdon et al.28 found that a lower CH was more associated with progression of visual field loss in their study than was a lower CCT.
It has only recently become possible to measure the biomechanical properties of the cornea in vivo, and the importance of these properties rests primarily with its effects on IOP measurement. It is possible, however, that corneal biomechanics may give an indication of the struc tural integrity of the optic nerve head. Further work is required to determine precisely how we might be able to risk-stratify glaucoma patients based on their bio me chan ical properties. OM
References
1. Goldmann H, Schmidt T. Uber applanationstonometrie. Ophthalmologica. 1957; 134:221-42.
2. Ehlers N, Hansen FK, Aasved H. Biometric correlations of corneal thickness. Acta Ophthalmol (Copenh). 1975;53:652-9.
3. Ehlers N, Hansen FK. Central corneal thickness in low-tension glaucoma. Acta Ophthalmol (Copenh). 1974;52:740-6.
4. Von Bahr G. Measurements of the thickness of the cornea. Acta Ophthalmol. 1948;26:247-266.
5. Von Bahr G. Corneal thickness; its measurement and changes. Am J Ophthalmol. 1956;42:251-266.
6. Brubaker R F. Tonometry and corneal thickness. Arch Ophthalmol. 1999;117: 104-05.
7. Liu J, Roberts CJ. Influence of corneal biomechanical properties on intraocular pressure measurement: quantitative analysis. J Cataract Refract Surg. 2005; 31:146-155.
8. Luce DA. Determining in vivo biomechanical properties of the cornea with an ocular response analyzer. J Cataract Refract Surg. 2005;31:156-162.
9. Kotecha A, Elsheikh A, Roberts CR, et al. Corneal thickness- and age-related biomechanical properties of the cornea measured with the ocular response analyzer. Invest Ophthalmol Vis Sci. 2006;47: 5337-5347.
10. Hayes S, Boote C,Tuft SJ, et al. A study of corneal thickness, shape and collagen organisation in keratoconus using videokeratography and X-ray scattering techniques. Exp Eye Res. 2007;84:423-434.
11. Meek KM, Leonard DW,Connon CJ, et al. Transparency, swelling and scarring in the corneal stroma, Eye. 2003;17: 927-936.
12. Kirwan C, O'Keefe M, Lanigan B. Corneal hysteresis and intraocular pressure measurement in children using the reichert ocular response analyzer, Am J Ophthalmol. 2006;142: 990-92.
13. Pepose JS, Feigenbaum SK, Qazi MA, et al. Changes in corneal biomechanics and intraocular pressure following LASIK using static, dynamic, and noncontact tonometry, Am J Ophthalmol. 2007;143:39-47.
14. Sanchez-Thorin JC. The cornea in diabetes mellitus. Int Ophthalmol Clin. 1998; 38:19-36.
15. Monnier VM, Sell DR, Abdul-Karim FW, et al. Collagen browning and cross-linking are increased in chronic experimental hyperglycemia: relevance to diabetes and aging. Diabetes. 1988;37:867-872.
16. Wollensak G, Spoerl E, Seiler T. Stress-strain measurements of human and porcine corneas after riboflavin-ultraviolet-A-induced cross-linking. J Cataract Refract Surg. 2003;29:1780-85.
17. Dupps WJ, Netto MV, Herekar S, et al. Surface wave elastometry of the cornea in porcine and human donor eyes. J Refract Surg. 2007;23:66-75.
18. Krueger RR, Ramos-Esteban JC. How might corneal elasticity help us understand diabetes and intraocular pressure? J Refract Surg. 2007;23:85-88.
19. Goldich Y, Barkana Y, Gerber Y, et al. Effect of diabetes mellitus on biomechanical parameters of the cornea. J Cataract Refract Surg. 2009;35:715-19.
20. Brandt JD, Beiser JA, Kass MA, et al. Central corneal thickness in the OHTS. Ophthalmology. 2001;108:1779-1788.
21. LaRosa FA, Gross RL, Orengo-Nania S. Central corneal thickness of Caucasians and African Americans in glaucomatous and nonglaucomatous populations. Arch Ophthalmol. 2001;119:23-7.
22. Nemesure B, Wu S Y, Hennis A, et al. Corneal thickness and intraocular pressure in the Barbados eye studies. Arch Ophthalmol. 2003;121:240-44.
23. Shimmyo M, Ross AJ, Moy A, et al. Intraocular pressure, Goldmann applanation tension, corneal thickness, and corneal curvature in Caucasians, Asians, Hispanics, and African Americans. Am J Ophthalmol. 2003;136:603-13.
24. Henderson PA, Medeiros FA, Zangwill LM, et al. Relationship between central corneal thickness and retinal nerve fiber layer thickness in ocular hypertensive patients. Ophthalmology. 2005;112:251-56.
25. Wells A, Garway-Heath D, Poostchi A, et al. Corneal hysteresis but not central corneal thickness correlates with optic nerve surface deformation in glaucoma patients. Invest Ophthalmol Vis Sci. 2008;49:3262-68.
26. Quigley HA, Brown A, Dorman-Pease ME. Alterations in elastin of the optic nerve head in human and experimental glaucoma. Br J Ophthalmol. 1991;75:552-57.
27. Hernandez MR. Ultrastructural immunocytochemical analysis of elastin in the human lamina cribrosa. Changes in elastic fibers in primary open-angle glaucoma. Invest Ophthalmol Vis Sci. 1992;33:2891-2903.
28. Congdon NG, Broman AT, Bandeen-Roche K, et al. Central corneal thickness and corneal hysteresis associated with glaucoma damage. Am J Ophthalmol. 2006;141:868-875.
![]() |
Leon W. Herndon MD, is associate professor of ophthalmology/glaucoma service at the Duke University Eye Center in Durham, NC. He can be reached via e-mail at hernd012@mc.duke.edu. |