Prospects for Continuous IOP Monitoring Devices
Will we ever have a patient-friendly way to monitor IOP "around the clock"?
By Arthur J. Sit, MD
Ophthalmologists have oriented a good deal of our glaucoma management strategies around lowering intraocular pressure, as it is the only modifiable risk factor at our disposal. Unfortunately, it is a rather imprecise biomarker of disease. Variations of IOP in humans have been known for at least a century, with fluctuations occurring in a regular pattern as a circadian rhythm, or randomly over short and long periods. However, current management of glaucoma only involves periodic measurements of IOP during office hours. This is a suboptimal approach, as it provides an incomplete description of the variable nature of intraocular pressure.
Devices capable of monitoring IOP around the clock would improve our ability to understand the dynamic processes at work in glaucoma. This review, adapted from a paper appearing in April/May 2009 Journal of Glaucoma, examines the progress toward continuous pressure monitoring as a method of obtaining more complete IOP profiles.
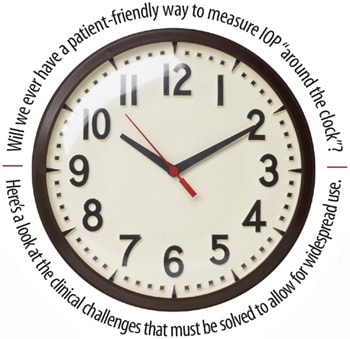
Why Continuous IOP Monitoring?
Although diurnal variation in IOP has been studied since Drance's early work in the 1960s, our thinking on the course it follows has changed in recent years. Initially it was believed that IOP is highest in the morning and declines during nocturnal hours, but such measurements did not consider the changes in body position that occur during sleep. More recent studies demonstrated that circadian IOP measured in the appropriate physiologic positions (sitting while awake and supine while asleep) was significantly higher during the nocturnal period than the diurnal period. A 2005 study found that two-thirds of glaucoma patients and over 90% of healthy controls had IOP peaks nocturnally.1
This nocturnal elevation in IOP, combined with the drop in systemic blood pressure that normally occurs during sleep, may compromise optic nerve head perfusion in susceptible individuals, and such patients may be more susceptible to disease progression.2 Normotensive glaucoma disease severity has also been associated with circadian variations in mean ocular perfusion pressure.3 Exacerbating this problem is the finding that not all pressure-lowering treatments are effective at night.
Nocturnal IOP elevations may be fundamental to understanding and managing glaucomatous progression in some cases. However, nocturnal pressures are difficult to obtain, requiring overnight admission of a patient into a sleep laboratory or in-patient facility for periodic pressure measurements. As well, the need to awaken patients for nocturnal IOP measurements has created some controversy concerning their accuracy in representing sleeping IOP. No currently available technology permits IOP measurements in sleeping patients.
Daytime IOP measurements have shortcomings as well. IOP may fluctuate randomly from exam to exam, making it difficult for clinicians to distinguish normal fluctuation from disease progression or response to therapy. Measuring IOP at different times in a single day to map a diurnal pressure curve is labor intensive and typically will represent only a portion of the true 24-hour IOP pattern. Tonometry readings themselves are susceptible to sources of error such as attempted eyelid closure, hypofluorescence of the pre-corneal tear film, accommodation, Valsalva maneuver, eye position and body habitus.
Increasing the frequency of clinic IOP measurements cannot overcome these problems, but automated ambulatory continuous IOP measurement may be able to do so.
Continuous IOP monitoring is a strategy to automatically measure IOP over the 24-hour period, using either a noninvasive monitor for a temporary period (e.g., 24 to 48 hours) or a permanent implantable pressure sensor coupled with an external data collection system. The former concept may be suitable for diagnosis and assessment of therapy for mild to moderately advanced glaucoma patients, while the latter would be appropriate for patients with advanced glaucomatous optic neuropathy or those already being considered for surgery. Each is discussed below.
Permanent IOP Monitoring
Concepts for an implantable permanent IOP monitor date back to the late 1960s, when Collins4 described a device using a capacitive circuit consisting of a pair of parallel spiral coils encased within a gas-filled plastic pill. As the pressure in the eye changed, the distance between the coils changed due to compression of the gas bubble, resulting in a change in the capacitance and resonant frequency of oscillation. An external sensor could detect oscillations in the coils to record IOP changes. However, questions about the stability and proper positioning of the device limited further development.
Rethinking the concept in the early 1990s, Svedbergh5 envisioned incorporating the pressure sensor into an intraocular lens, made possible by advances in miniaturization. In vitro testing was promising, but issues concerning variable signal strength and measurement noise remained. Those obstacles were recently addressed by a new design6 that made use of microfabrication of components. Although this approach seems promising based on animal testing, results of human trials have not been reported to date.
Other concepts proposed include measuring IOP fluctuations by incorporating strain gauges into a silicone band scleral buckle7 and techniques to continuously record IOP via telemetry after implantation of commercially-available pressure monitors.8,9 However, the invasive nature of such devices make them impractical for clinical use.
Currently, none of the proposed devices for permanent pressure monitoring have reported human trials, but characteristics of a suitable device can be deduced from previous attempts. It would need to be biocompatible, powered wire-lessly and maintain a stationary position with respect to the eye.
As a permanent pressure monitor would likely reside intraocularly, it may potentially provide the most reliable IOP measurements of any method, as it would not be influenced by mechanical properties of the eye such as corneal thickness. Long-term safety issues and questions about the ideal location for placement of such a device remain. Current developments would seem to favor the anterior chamber or capsular bag in the case of the IOL-based monitor. However, the vitreous has also been used, and one recent proposal has been to place a transducer at the surface of the choroid.10
The ideal time interval between measurements is also unclear, but even measurements every few minutes would be a marked improvement over current clinical practice. Also yet to be fully understood is the potential impact of ocular and body movements on IOP readings.
Lastly, and perhaps most importantly, the indications for permanent IOP monitoring need to be clarified. The requirement for surgical implantation would likely limit the use of permanent IOP monitors to high-risk patients or those already undergoing surgery such as cataract extraction or filtration procedures. As well, the clinical utility of permanent continuous IOP monitoring would likely need to be demonstrated before such devices can gain regulatory approval and widespread usage. Acquiring this knowledge may require the development of temporary noninvasive IOP monitoring.
Temporary IOP Monitoring
As with permanent monitoring, temporary IOP monitoring is not a new idea. In 1958 Maurice11 gave the first description of such a device: an automated recording indentation tonometer mounted on the forehead and held in place on the eye with a headband. Although it did allow some degree of ambulation, it was clearly not intended for clinical use. Even for research, the duration of IOP recordings were limited to about 20 minutes based on the ability of the wearer to maintain fixation.
Nissen12 tested an “applanating suction cup” — essentially an applanation tonometer held in place by a suction ring — that estimated IOP by measuring the pressure of the fluid interface between the device and the cornea instead of measuring the force required for applanation. As such, a constant infusion of saline to the interface region was required. The need for lid speculums and frequent use of topical anesthetic, plus restriction of eye movement, limited its role to primarily research tool; it could not be used for human measurement for more than 60 minutes.
With the goal of producing a clinical tool, Cooper et al.13 developed a device they called a scleral guard ring that used a capacitive pressure sensor similar in principle to the type described by Collins4 for intraocular use. However, instead of measuring pressure internally, the device was held against the scleral surface, causing an applanation. Changes in IOP would result in deformation of the device with subsequent changes in capacitance and resonant frequency that could be detected externally. However, there were persistent problems with moisture in the device and difficulty maintaining coupling to the sclera. More fundamentally, the sclera's variable viscoelastic properties confounded accurate assessment of IOP.
Cornea is also a viscoelastic material, but tends to be less variable than sclera, making it a more suitable site for IOP monitoring. As early as 1974, Greene and Gilman14 developed silicone contact lenses with embedded strain gauges to measure the change in the meridional angle of the cor-neoscleral junction with variation in IOP. The contact lenses, however, needed to be custom molded for individual eyes to minimize artifactual movement of the strain gauges and the technology of the time required wire leads for power and output recording.
An update of this concept uses soft contact lenses to detect changes in the radius of curvature of the cornea with IOP.15 Studies with enucleated porcine eyes demonstrated high sensitivity, but results may be vulnerable to significant motion artifacts. Recently, this group presented results of short-term human trials using a wireless version of the device.16 The authors reported the ability to filter out noise to detect an artificially-induced intraocular pressure change over a 10-minute interval. The device is currently available in Europe as the Triggerfish system (Sensimed AG, Lausanne, Switzerland) but is not FDA approved. Although promising, longer-term trials remain to be performed, along with the validation of the ability to discern true IOP changes from normal variations in corneal radius of curvature.
To succeed clinically, a temporary pressure monitoring device will require noninvasive external measurements and a design that is self- or wirelessly powered (or that requires no power). It would also need to be biocompatible and comfortable enough for most patients to wear at least 24 hours. Noise and artifactual IOP readings must also be avoided or compensated for in some way.
Another issue is the problem of IOP measurement through eyelids. The ocular surface environment is significantly different in terms of surface tension, temperature and light exposure when the eyes are closed compared with when the eyes are open. Any system performing continuous external IOP measurements will need to cope with these variable conditions.
Forthcoming Challenges
The technologic capability for permanent continuous IOP monitoring certainly exists at this time, and is used in laboratory animals routinely with great success. It also appears that devices suitable for human use will be available in the near future. However, regulatory approval and widespread adoption of any such technology will likely need proof of clinical utility. This presents a quandary, as continuous IOP monitoring devices need to be first developed to demonstrate their usefulness.
Temporary continuous IOP monitoring may prove to be the technology that is required to provide this information. Unfortunately, noninvasive temporary pressure monitors may have greater technical challenges related to the need for external IOP measurements. The first temporary continuous IOP monitoring systems are starting to appear in the market but further investigation is required to evaluate their potential. The development of this technology will be a critical step in understanding and managing IOP variations in glaucoma. OM
References
- Mosaed S, Liu JH, Weinreb RN. Correlation between office and peak nocturnal intraocular pressures in healthy subjects and glaucoma patients. Am J Ophthalmol. 2005;139:320-324.
- Graham SL, Drance SM. Nocturnal hypotension: role in glaucoma progression. Surv Ophthalmol. 1999;43(suppl 1):S10-S16.
- Choi J, Kim KH, Jeong J, et al. Circadian fluctuation of mean ocular perfusion pressure is a consistent risk factor for normal-tension glaucoma. Invest Ophthalmol Vis Sci. 2007;48:104-111.
- Collins CC. Miniature passive pressure transensor for implanting in the eye. IEEE Trans Biomed Eng. 1967;14:74-83.
- Svedbergh B, Backlund Y, Hok B, et al. The IOP-IOL. A probe into the eye. Acta Ophthalmol (Copenh). 1992;70:266-268.
- Schnakenberg U, Walter P, Bogel GV, et al. Initial investigations on systems for measuring intraocular pressure. Sens Actuators A Phys. 2000;85:287-291.
- Wolbarsht ML, Wortman J, Schwartz B, et al. A scleral buckle pressure gauge for continuous monitoring of intraocular pressure. Int Ophthalmol. 1980;3:11-17.
- McLaren JW, Brubaker RF, FitzSimon JS. Continuous measurement of intraocular pressure in rabbits by telemetry. Invest Ophthalmol Vis Sci. 1996;37:966-975.
- Schnell CR, Debon C, Percicot CL. Measurement of intraocular pressure by telemetry in conscious, unrestrained rabbits. Invest Ophthalmol Vis Sci. 1996;37:958-965.
- Rizq RN, Choi WH, Eilers D, et al. Intraocular pressure measurement at the choroid surface: a feasibility study with implications for implantable microsystems. Br J Ophthalmol. 2001;85:868-871.
- Maurice DM. A recording tonometer. Br J Ophthalmol. 1958;42:321-335.
- Nissen OI. Continuous recording of the intraocular pressure in human and rabbit eyes with a simple applanating suction cup. Acta Ophthalmol. 1977;55:750-760.
- Cooper RL, Beale D. Radio telemetry of intraocular pressure in vitro. Invest Ophthalmol Vis Sci. 1977;16:168-171.
- Greene ME, Gilman BG. Intraocular pressure measurement with instrumented contact lenses. Invest Ophthalmol. 1974;13:299-302.
- Leonardi M, Leuenberger P, Bertrand D, et al. First steps toward noninvasive intraocular pressure monitoring with a sensing contact lens. Invest Ophthalmol Vis Sci. 2004;45:3113-3117.
- Pitchon EM, Leonardi M, Renaud P, et al. First in Vivo Human Measure of the Intraocular Pressure Fluctuation and Ocular Pulsation by a Wireless Soft Contact Lens Sensor. Association for Research in Vision and Ophthalmology. FL: Fort Lauderdale; 2008.
Arthur J, Sit, SM, MD, is assistant professor of ophthalmology at the Mayo Clinic College of Medicine in Rochester, MN. He can be reached at sit.arthur@mayo.edu. |